A: GNSS users who must verify the integrity of their navigation solutions to meet safety requirements need at least some knowledge of the degree to which they can trust the signals received from GNSS satellites before additional monitoring is added.
By Sam Pullen, Todd Walter, Juan Blanch, Rebecca Wang, Stanford University
The “GNSS Solutions” column in the January/February 2021 issue of Inside GNSS [1] described in some detail the commitments made for the Global Positioning System (GPS) in the GPS Standard Positioning Service Performance Standard (GPS SPS PS) [2]. However, two questions remain. First, how can the numerical values within these commitments be updated if needed between updates of the GPS SPS PS, or similar documents issued by other service providers (e.g., Galileo, GLONASS, Beidou)? Second, what commitments have been made by other GNSS service providers?
This column will address these questions with a focus on the parameters related most directly to integrity verification.
Need for Service Provider Commitments
The example of the GPS SPS PS has motivated other GNSS service providers to issue “service definition” documents [3] with similar purposes—basically, to define the key parameters of each service provided by a given GNSS constellation to the point where users can model the performance that it will provide for their applications and have at least some degree of reliance that the service will be maintained with these parameters (or better). Because these constellations are not yet fully mature and do not have the decades of service history that GPS does, the performance commitments in these documents tend to be less specific.
Unlike augmentation systems such as SBAS and GBAS, Advanced Receiver Autonomous Integrity Monitoring, or ARAIM, is not dependent on real-time reception of differential corrections and integrity flags from external sources. As a result, detailed knowledge of the inherent integrity of received GNSS satellite signals is of greater importance to ARAIM, although it is also important to the design and validation of augmentation systems. While SBAS and GBAS currently augment GPS only and can make use of the detailed information provided in [2], most ARAIM applications will combine satellites from multiple GNSS constellations and need trustworthy information about each of the constellations whose signals are used.
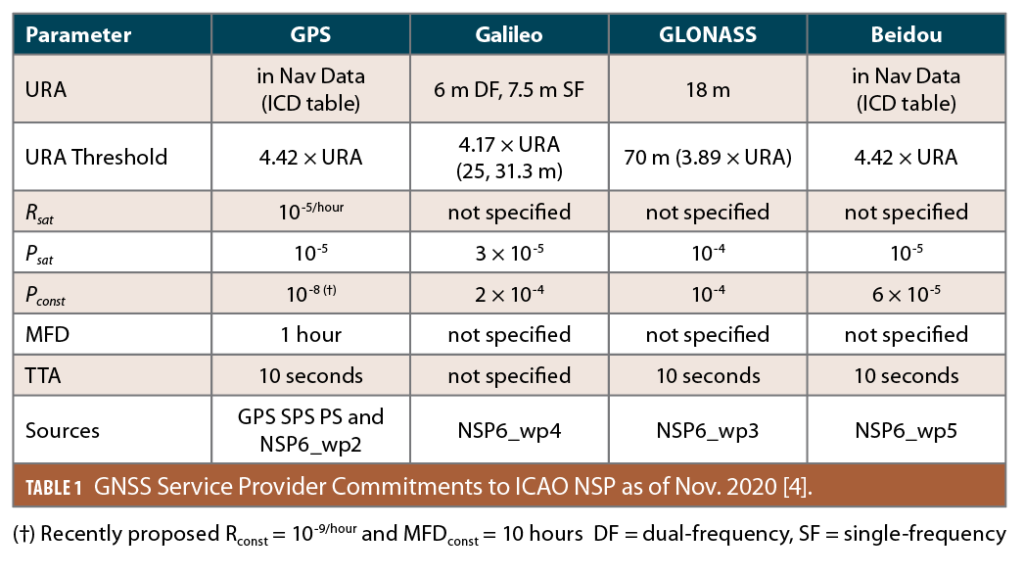
ICAO Service Provider Commitments and Definitions
Development of ARAIM within the civil aviation community led to an effort within the International Civil Aviation Organization (ICAO) Navigation Systems Panel (NSP) to obtain additional integrity-related commitments from GNSS service providers. Table 1 (from [4]) shows a summary of the results obtained through this process based on working papers submitted to the ICAO NSP-6 meeting in November 2020. The parameters listed in the left-hand column are defined as follows [5, 6]:
URA (User Range Accuracy): In the integrity context, this is a one-standard-deviation (“sigma”, or σ) value of Signal-in-Space (SIS) range errors chosen such that a zero-mean Gaussian distribution with this standard deviation bounds the distribution of actual range errors in this category (e.g. not including user receiver or local user environment errors) to the probability specified by Psat. In other words, a zero-mean Gaussian distribution with σ = URA conservatively covers nominal SIS errors, whereas errors with probabilities below Psat are treated as anomalous (or faulted) and are not described by this distribution. Note this definition of URA is more conservative than the similar parameter URE, or User Range Error, which also expresses typical range errors at the one-sigma level but is not meant to bound errors out to Psat (thus, URA > URE).
URA Threshold: This defines the SIS error level beyond which errors are considered anomalous or faulted as opposed to nominal. This is typically cited as a multiple of URA, and when URA is a fixed value (as for Galileo and GLONASS in Table 1), the URA Threshohold is also a constant.
Rsat: This is a conservative bound on the per-satellite failure rate per unit of time for failures that are statistically independent across satellites of the same constellation. It represents the probability that a new failure will occur on an otherwise-healthy satellite in the next time period specified (e.g., faults per hour).
Psat: This is a conservative bound on the per-satellite failure state probability for statistically independent satellite failures. It represents the probability at any given time that a satellite will be in a faulty (as opposed to nominal) state and thus is unitless. The baseline ARAIM algorithm developed for civil aviation [6] uses this probability as an input, whereas satellite failure probabilities are more typically given as rates.
Note that, in Table 1, the values of Psat correspond to the multipliers of URA in a two-sided zero-mean Gaussian distribution. For example, for GPS, 4.42 corresponds to a probability of exceedance of 10-5 in a two-sided standard Gaussian distribution. This is necessary because, as explained earlier, the URA threshold represents the size of error above which a faulted state is deemed to exist, and this must be consistent with the probability of a faulted state given the underlying assumption of a zero-mean Gaussian distribution with σ = URA.
Pconst: This is a conservative bound on the state probability of failures correlated across satellites in the same constellation, including faults of two or more up to all satellites in that constellation. This is of particular concern to ARAIM because correlated faults affecting an entire constellation can only be detected by comparing to other (non-faulted) GNSS constellations. Note that constellation failure rates (Rconst) are not specified except for GPS, and the value for GPS in Table 1 is not yet included in [2].
MFD (Mean Fault Duration, also known as Mean Time to Notify, or MTTN): This is an estimate of the mean duration of a fault from the onset of a fault state to its resolution, which is typically when civil users are alerted by the affected constellation to no longer use that satellite for positioning. It is used to convert probability rates (R) into state probabilities (P) (and vice versa, if needed) using the approximation P = MFD × R.
Note that P is unitless, whereas MFD is in units of time and R is in units of 1/time.
TTA (Time to Alert): This is the maximum interval between the time at which a faulted condition begins (based on range errors exceeding the URA Threshold) and when it must end or be alerted to prevent it from being considered a fault from the point of view of potential integrity loss. For example, because TTA is given as 10 seconds for all but Galileo satellites in Table 1, range error growth on a single GPS, GLONASS or Beidou satellite that exceeds the URA Threshold at an arbitrary time t0 only “counts” as a fault (from the perspective of Psat and Rsat) if the range error continues to exceed the URA Threshold and be usable past t0 + 10 seconds.
Differences Among GNSS Constellations
The differences among the parameter commitments across constellations in Table 1 are due to many factors. One of these is the maturity of GPS and its decades of reliable performance compared to the relatively new and evolving nature of Galileo and Beidou and the many changes that have occurred within GLONASS. The GPS SPS PS [2] that is the basis for the GPS numbers in Table 1 is the fifth edition of a document that dates back to the declaration of GPS Initial Operational Capability (IOC) in late 1993, whereas the most equivalent Galileo document (the OS SDD [3]) is Issue 1.2 of a document that dates back to late 2016. The greater maturity of GPS is the primary reason the other constellations do not provide specific values for all parameters listed in Table 1.
Three parameters for which values are provided by all constellations are URA, Psat, and Pconst, and these are worth comparing. Starting with Psat, state fault probabilities from 10-4 (GLONASS) to 3 × 10-5 (Galileo) are provided. The value of 10-5 provided for GPS is known to be conservative because only five GPS single-satellite faults are known to have occurred since 2008 (and only one since 2012) [6,7]. Because data for fewer years is available for the current versions of the other constellations, and multiple failures have occurred on both Galileo and GLONASS in recent years, their Psat values are at least as large or larger. Regarding correlated or constellation failures, none of these are known to have occurred on GPS in its history (back to achievement of GPS Full Operational Capability or FOC in mid-1995), thus GPS can provide a much lower value of Pconst than the other constellations [7].
Examining URA, two different approaches are shown in Table 1. GPS and Beidou promise the URA values included in their most recent broadcast navigation data for each satellite meet the definition of URA and the URA Threshold, meaning their application in a zero-mean Gaussian distribution bounds range errors out to Psat. In contrast, Galileo and GLONASS only commit to fixed URA values, not the error estimates included in their navigation data. In other words, Galileo and GLONASS are (at least for now) declining to promise that their broadcast error values (such as the Galileo Signal in Space Accuracy (SISA) parameter [3]) sufficiently bound nominal errors. Because the fixed URA values promised instead must exceed those that could be broadcast by healthy satellites, they are significantly larger and more conservative.
Figures 1 and 2 show how these different approaches affected the error bounds and bounding margins provided by GPS and Galileo, respectively, in 2021 [8]. Figure 1 shows the complementary cumulative distribution function (CCDF, or 1 minus CDF) of instantaneous GPS satellite user range errors (IURE) in 2021 (by individual satellite and over all satellites) normalized by the broadcast URA from the GPS legacy navigation data (LNAV) at the time of each measurement. The LNAV URA is most commonly 2.4 meters but can occasionally increase to larger values depending on the GPS Control Segment’s assessment of the accuracy of its broadcast clock and ephemeris parameters. The CCDF of a standard Gaussian distribution is shown in red to demonstrate that it bounds (in a CDF sense) the error distributions of each satellite. Most GPS satellites have errors at probabilities of 10-4 to 10-5 (representing Psat) that are much lower than the bounding Gaussian curve, and the few satellites with larger errors at these probabilities are still bounded with margin.
Figure 2 shows the same plot for the Galileo constellation except the normalization by URA is based on the fixed 6-meter value for dual-frequency users in Table 1 rather than the broadcast SISA values. Except for the two Galileo satellites that experienced failures in 2021 (SVs 102 and 210) [8], the standard Gaussian curve greatly exceeds the satellite error CCDFs. This demonstrates both the low level of nominal errors in Galileo generally as well as the conservatism in the use of a fixed value of 6 meters for URA. The broadcast SISA is most commonly set to 3.1 meters, and if this value were used for normalization, the red Gaussian curve would still bound all of the satellite CCDFs with margin except for the two that experienced failures.
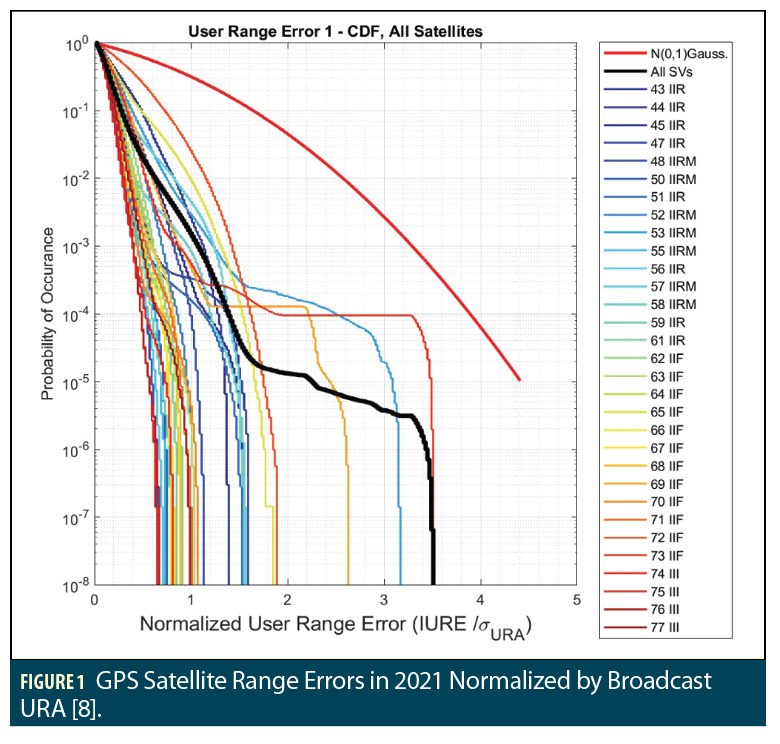
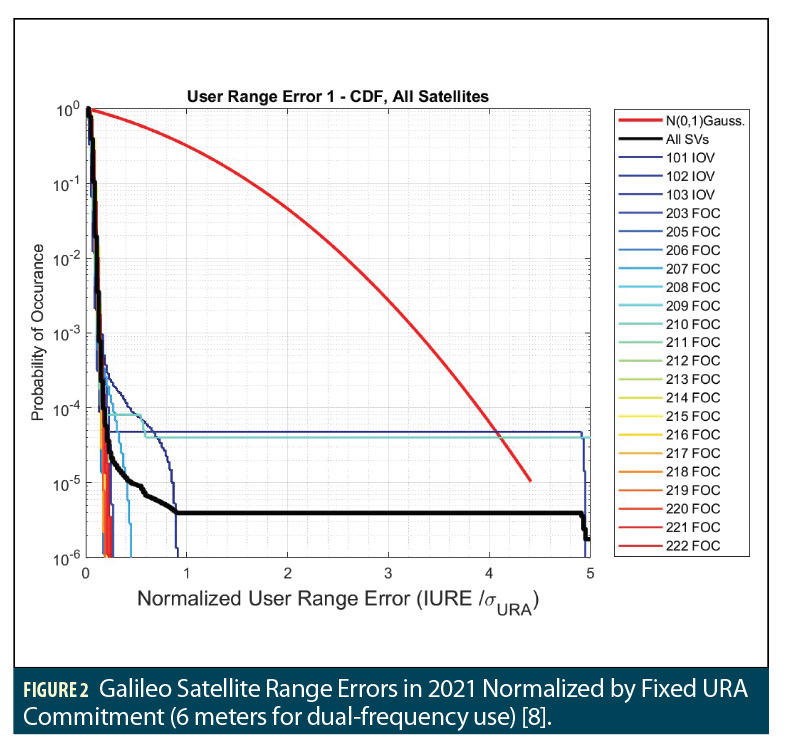
Use in Augmentation Systems and ARAIM—The Role of the ISM
GPS augmentation systems such as SBAS and GBAS were developed and certified before the current GPS constellation commitments were obtained based on earlier versions of the GPS SPS PS and interactions with the GPS Control Segment to confirm (to the extent possible) that SBAS and GBAS assumptions (“assertions”) regarding GPS performance and integrity were reasonable. However, the commitments offered for other GNSS constellations support the development of modernizations to SBAS, GBAS and other GNSS augmentations that combine signals from multiple constellations.
ARAIM, on the other hand, is directly dependent on the integrity commitments made by GNSS service providers, as it relies on these values to calculate the protection levels that provide real-time integrity assurance to users [5]. The criticality of potential errors in these commitments (such as underestimates of the true URA or Psat) depends on the application to which ARAIM is applied. The initial implementation of ARAIM for civil aviation will support 2D horizontal navigation only (“H-ARAIM”) and thus represents a replacement and enhancement of the more traditional forms of RAIM commonly in use. The phases of flight that H-ARAIM will support are of “Major” severity should integrity be lost, and existing RAIM is normally not used as the sole means of guidance. Therefore, the commitments offered by GNSS service providers may be sufficient for H-ARAIM if they are actively monitored by the providers and updated (if necessary) via issuance of revised commitments to ICAO.
Once H-ARAIM is placed into operation and shown to be successful, ARAIM could be extended to support vertical and horizontal navigation during approaches to 250 or 200 ft decision heights under instrument flight conditions (“V-ARAIM”). The severity of loss of integrity for these operations is “Hazardous,” which allows much less margin for both erroneous committed values and conservatism in these values (because excess conservatism will limit the availability of V-ARAIM). As a result, some means of updating or re-issuing these values on a regular basis is needed. To allow this, several concepts for an ARAIM Integrity Support Message (ISM) have been proposed, such as messages included in GPS or Galileo navigation data or messages provided via another aviation datalink. The specific data fields to be included in an ISM and the frequency with which they would be updated (if required) have been studied for some time and remain under evaluation.
Summary and Ongoing Work
This article introduces the integrity-related parameter commitments made recently by four different GNSS constellation service providers to civil aviation users of GNSS via ICAO. Detailed definitions of the relevant parameters are provided, and comparisons of the values provided among the various constellations are shown. The importance of these commitments to safety-of-life applications of GNSS is described with a focus on H-ARAIM and V-ARAIM, and the need for regular updates or at least re-assurances of these parameters via an Integrity Support Message (ISM) is discussed.
Although some form of ISM is planned for V-ARAIM, it is unclear whether its contents would or could be updated more often than once or twice per year or if the basis for the broadcast parameter values would go beyond the existing monitoring performed internally by each GNSS constellation (which supports the current commitments).
One aspect of ongoing research on V-ARAIM is the degree to which more rapid ISM updates (weekly, daily or even hourly) combined with additional monitoring of each constellation would allow the key ISM parameters to be improved and thus support higher availability. This is of particular importance for users like the U.S. military, who may be constrained to use the GPS Precise Positioning Service (PPS) along with (at most) the Galileo Open Service in any future ARAIM implementation. Results of this work are shown in [4] and will be described in a future GNSS Solutions column.
References
(1) “GPS Solutions” column, Inside GNSS, Vol. 16, No. 1, Jan./Feb. 2021, pp. 18-23. https://lsc-pagepro.mydigitalpublication.com/publication/?m=61061&i=690698&p=18&ver=html5.
(2) GPS Standard Positioning Service (SPS) Performance Standard (GPS SPS PS), Washington DC, U.S. Dept. of Defense, 5th Edition, April 2020. https://www.gps.gov/technical/ps/2020-SPS-performance-standard.pdf.
(3) Galileo—Open Service—Service Definition Document (Galileo OS SDD), European Union Agency for the Space Programme (EUSPA), Issue 1.2, Nov. 2021. https://www.gsc-europa.eu/sites/default/files/sites/all/files/Galileo-OS-SDD_v1.2.pdf.
(4) S. Pullen, S. Lo, et al, “Ground Monitoring to Support ARAIM for Military Users: Alternatives for Rapid and Rare Update Rates,” Proceedings of ION GNSS+ 2021, St. Louis, MO., Sept. 2021. http://web.stanford.edu/group/scpnt/gpslab/pubs/papers/Pullen_IONGNSS_2021_Mil_ARAIM.pdf.
(5) J. Blanch, T. Walter, et al., “Baseline advanced RAIM user algorithm and possible improvements,” IEEE Transactions on Aerospace and Electronic Systems, Vol. 51, No. 1, Jan. 2015, pp. 713-732. https://doi.org/10.1109/TAES.2014.130739.
(6) T. Walter, J. Blanch, et al., “Development of Fault Probabilities for ARAIM,” IEEE Transactions on Aerospace and Electronic Systems, Vol. 55, No. 6, Dec. 2019, pp. 3505-3516. https://doi.org/10.1109/TAES.2019.2909727.
(7) J. Blanch, X. Liu, et al., “Analysis of GNSS Constellation Performance for Advanced RAIM,” Proceedings of ION GNSS+ 2021, St. Louis, MO., Sept. 2021. http://web.stanford.edu/group/scpnt/gpslab/pubs/papers/Blanch_IONGNSS_2021_ARAIM_Mon.pdf.
(8) R. Wang, “Characterization of GNSS Signals for Safety of Life Navigation,” Dept. of Aeronautics and Astronautics, Stanford University, Unpublished Presentation, May 2022.
Authors
Todd Walter is a Research Professor in the Department of Aeronautics and Astronautics at Stanford University. He is also a member of the National Space-Based Positioning, Navigation, and Timing (PNT) Advisory Board. His research focuses on implementing satellite navigation systems for safety-of-life applications. He has received the Institute of Navigation (ION) Thurlow and Kepler awards. He is also a fellow of the ION and has served as its president.
Juan Blanch is a senior research engineer at Stanford University, where he works on integrity algorithms for Space-based Augmentation Systems and on Receiver Autonomous Integrity Monitoring. A graduate of Ecole Polytechnique in France, he holds an MS in Electrical Engineering and a Ph.D. in Aeronautics and Astronautics from Stanford University. He received the 2004 Institute of Navigation (ION) Parkinson Award for his doctoral dissertation and the 2010 ION Early Achievement Award.
Rebecca Wang is a graduate student in the GPS Research Laboratory working under the guidance of Professor Todd Walter in the Department of Aeronautics and Astronautics at Stanford University. Prior to joining the lab, Rebecca received her B.S. in Aerospace Engineering at the University of Texas at Austin. Her research interests include multi-GNSS integrity for aviation and high-accuracy navigation.