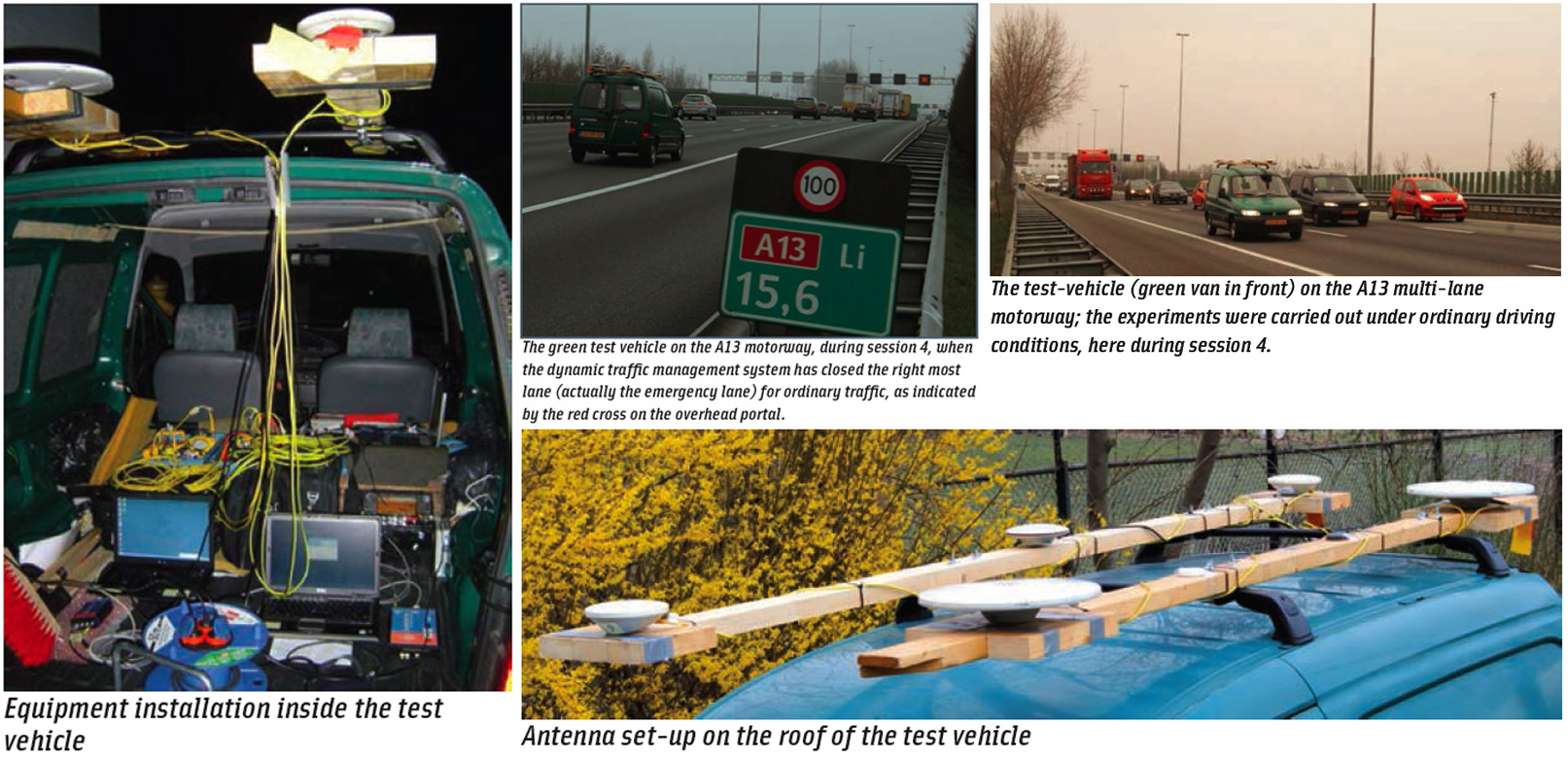
Next-generation car navigation may well require the ability to identify the lane in which a vehicle is operating on a motorway. This could support advanced driver assistance in general as well as the observation and study of driver behavior and traffic flow. Such road vehicle applications call for sub-meter positioning accuracy, often in real-time — all this preferably at low-cost.
Next-generation car navigation may well require the ability to identify the lane in which a vehicle is operating on a motorway. This could support advanced driver assistance in general as well as the observation and study of driver behavior and traffic flow. Such road vehicle applications call for sub-meter positioning accuracy, often in real-time — all this preferably at low-cost.
In this article we will investigate whether real-time single-frequency precise point positioning (RT SF-PPP) can meet the demands set for such applications. Precise point positioning can provide improved position accuracy, as compared to standalone GPS positioning, but without the need for local or regional differential GPS (DGPS)-like infrastructure.
Static and Post-Processed vs. Kinematic and Real-Time
Researchers at Delft University of Technology have developed a real-time version of SF-PPP, based on an algorithm that uses undifferenced single-frequency pseudorange code and carrier phase observations of the user receiver in the vehicle. By combining these observations with predicted satellite orbits and global ionospheric maps (GIMs) as well as real-time satellite clock estimates, a position solution is computed on an epoch-by-epoch basis. The result is truly kinematic, without (relying on) any modeling of the user receiver dynamics.
The performance of RT SF-PPP was extensively demonstrated for static applications in the work described in the articles by R. J. P. van Bree et alia (2009) and (2011) listed in the Additional Resources section near the end of this article. The position accuracy presented in the latter article shows a 95 percent error of about 0.30 meter in the horizontal directions, and 0.65 meter in the vertical.
These results were obtained using high-end GPS receivers. Mid-range receivers produced values that were still smaller than one meter in all directions.
These findings have led to the question: can SF-PPP deliver the position performance required for lane identification of a road vehicle, in real-time, and preferably with low-cost equipment? Low-cost refers here to a fairly simple, single-frequency GPS receiver, with a patch antenna, delivering pseudorange code and carrier phase observations, and a price on the order of US$100 or less.
Recently, in spring 2011, we conducted a real-world kinematic trial with a vehicle on a local highway. The multi-lane motorway (A13 — pictured in an accompanying photo, above right) used for the test is located between the cities of The Hague and Rotterdam, runs past Delft, and is one of the busiest traffic routes in the Netherlands.
The test was carried out under ordinary driving conditions. The purpose of the trial was to assess the position accuracy and to test specifically the ability to identify the lane the car is driving in.
For the test we installed low-end and mid-range receivers in rigid mounts on the test vehicle’s roof, next to several antennas of high-end GNSS equipment. The latter are employed to reconstruct an accurate (centimeter-level) ground-truth for the low-end and mid-range receivers.
The high-end dual-frequency receivers used post-processed differential carrier-phase GPS corrections from a nearby reference station, namely a permanent GNSS station at TU Delft’s GNSS observatory, with accurately known position coordinates in the International Terrestrial Reference Frame (ITRF2005). In this way, a highly accurate reference track was available for quantitative error analysis of the obtained PPP-results.
Single-Frequency Precise Point Positioning
The best position accuracy with SF-PPP is achieved when we can use GPS data products that are as precise as possible, that is, when we have access to the final satellite clocks and satellite orbits, ionospheric maps, and differential code biases (DCBs). These products, however, are only available to a user with a significant latency of a few days or even weeks after the measurement epoch, ruling out real-time operation.
In real-time operations, we must use predicted satellite orbits, predicted GIMs, and — in particular — real-time satellite clock estimates. For the latter, we used the Real-time Clock Estimation (RETICLE) products from the German Space Operations Center (GSOC)/German Aerospace Center (DLR), which have been shown to deliver comparable performance as that of the International GNSS Service (IGS) final products. The RETICLE system computes clock corrections for the entire GPS constellation in real-time, currently based on a world-wide network of 37 reference stations.
In addition to the RETICLE products, we use predicted GIMs and predicted DCBs from the Center for Orbit Determination in Europe (CODE) in Bern, in order to enable real-time SF-PPP. The predicted GIMs are provided in 24-hour batches.
In the experiment described here, measurements were collected in the vehicle, and the needed data products were logged — in parallel — in the office. The actual processing took place back in the office after the fact, but strictly re-playing the real-time situation. Only measurements up to the observation epoch are used (hence, filtering but no smoothing), as well as only those data products available at the very time of the observation.
We only used GPS signals with a five-degree satellite elevation cut-off angle. Statistical testing of the measurements was performed specifically on outliers and cycle slips.
Requirement for Lane Identification
In the Netherlands, one lane on a motorway is 3.50 meters wide, and for successful lane-identification, the cross-track (or lateral) coordinate of the position fix has to lie within this lane.
Assuming that a car is driving exactly in the middle of the lane and with the antenna mounted exactly on the lengthwise center-line of the car, the probability of correct lane identification can be evaluated by integrating the position coordinate error probability density function (which in our case we assumed to be normal) from –3.50/2 meters to +3.50/2 meters (i.e., a two-sided interval about zero, where zero represents the situation in which the estimated position coordinate coincides with the true position).
For a system of advanced traffic flow monitoring, which may also provide guidance instructions to enhance traffic flow, we set — as a first guess — the required probability of correct lane identification at 95 percent, meaning that the lane location of only 5 cars out of 100 is misidentified. This leads to a 95 percent error requirement on the (lateral) coordinate of 1.75 meters.
Equipment and Installation
As mentioned, we used three classes of equipment in this test. The low end was represented by a single-frequency (L1), 16-channel GPS receiver that, on the basis of measurement accuracy, we believed to be representative of a good automotive GPS-chip today. This receiver was connected to a suitable 30-decibel gain mobile GPS patch antenna.
For the mid-range equipment we used a single-frequency GNSS receiver, configured as a 16-channel GPS L1 receiver and connected to a relatively simple patch antenna; the equipment cost here was on the order of US$1,000.
The high-end equipment consisted of a 12-channel dual-frequency GPS receiver with a geodetic antenna that cost on the order of US$10,000. Actually two of these receivers were used on the car primarily to reconstruct the ground truth trajectories. (For purposes of this article, our discussion of results in later sections uses data from only one of the receivers – that in the front of the vehicle. However, the performance of the other one was very similar.)
All receivers collected data at a 10-hertz rate, and only single-frequency measurements (C1 and L1) from these receivers were used for the PPP processing. Accompanying photos (above right) show the equipment installed in the vehicle and the antennas mounted on its roof.
In addition to the receivers on the car, another high-end receiver was used as a reference station, located a few kilometers away at the TU Delft GNSS observatory. This reference receiver was connected to a choke-ring antenna.
All antennas were attached to a solid wooden bar, which was rigidly mounted on the vehicle. For the experiment described in this article, only the bar at right (in the photo, above right) was used. The patch antennas for the low-end and middle class receiver were situated in the middle of this bar, and the two geodetic antennas for the high-end receivers were at the ends of the wooden bar.
Test Set-Up
Figure 1 shows a 5.5-kilometer section of motorway selected for the trial, which stretched from entrance/exit 10 (Delft Zuid) to exit/entrance 11 (Berkel en Rodenrijs/Rotterdam Airport). We next defined four one-hour sessions during the day, considering satellite visibility and geometry, ionospheric activity, and traffic activity on the A13 motorway. These one-hour sessions are listed in Table 1.
During these sessions, the minimum number of satellites available above a cutoff angle of five degrees was 9, and the maximum ranged from 11 to 13.
The 5.5-kilometer section of motorway was driven forth and back, and during each one-hour session five loops of the section were completed, yielding a total driven distance of about 57 kilometers per session. All four sessions together provided 228 kilometers worth of measurements, and close to 150,000 measurement epochs (at 10 hertz).
While driving, the car changed lanes several times, and driving speeds varied between 75 km/h (typically imposed by busy traffic) and 105 km/h (obeying, more or less, the legal limit of 100 km/h on this stretch of motorway). The total average speed, which includes stopping at traffic lights at the exits and entrances, lay around 60 km/h.
Visual Impression of Results
In this section we will present the SF-PPP results at a “global” level, primarily through visual inspection and an analysis of position solution availability.
Figures 2 and 3 present examples (snapshots) of trajectory overlays on aerial images, and thereby provide a first general impression of the SF-PPP positioning performance. These two figures represent all the position solutions of all three receivers from session 3.
During this session five circuits of this stretch of motorway were driven; so, for each color there will be five dots on either side of the road. Measurements were collected at the rate of 10 position updates per second.
In Figures 2 and 3 the green dots represent positions determined by the low-end GPS receiver, red dots for the mid-range receiver, and yellow for the high-end receiver. Usage of different lanes can be clearly distinguished from the trajectories produced by all three receivers, and in Figure 3 a change from the middle to the far left lane can be properly observed.
Table 2 presents data on the availability of the SF-PPP positioning results, which always exceeded 99 percent of the total number of (theoretically) available epochs.
Measurement data can be lost due to obstruction of the satellite signal by road infrastructure and other vehicles. This motorway typically has tall lampposts every 50 meters, and on this stretch there are 11 overhead traffic portals, of which 9 “light” ones carry electronic signs for dynamic traffic management. (A shadow of one of these signs is visible in Figure 2.) The other 2 “heavy” traffic portals carry huge, traditional road and destination signs. And directly next to the motorway, there are trees and vegetation every now and then.
Also large road vehicles such as trucks can block signals from several satellites during the tracking. And finally the quality of measurement data may be too poor to use: after detection of cycle slips and removal of outliers, too few measurements to satellites remain to be able to compute a position solution.
Kinematic Results
Centimeter-level, ground-truth trajectories have been computed (using dual-frequency differential carrier phase GPS with respect to a nearby reference station) and reconstructed for all of the receivers. Specifically, we calculated the trajectories for a stretch of the kinematic portion of the vehicle trials (one northbound drive from entrance 11 to exit 10 on the motorway, amounting to about four minutes of data), for each of the four sessions.
The receivers’ position errors are obtained by differencing the SF-PPP results with the ground truth trajectories. Position accuracy is then presented in terms of the 95th percentile (about zero) for each of the three coordinate components, north (N), east (E), and up (U). Figure 4 presents the results for the three types of receivers tested.
Little difference in performance appears between the mid-range (with patch antenna) and high-end receivers. The low-end receiver’s SF-PPP results are generally a factor 2–3 times worse when looking at the 95-percent figures, in particular in the Up-component. All receivers seem to meet the 1.75–meter (95%) requirement on the horizontal coordinates.
As noted earlier, both the low-end and mid-range receivers used a patch antenna. Nevertheless differences can be observed between the results of them. The low-end receiver likely uses (close to) the minimum bandwidth (two megahertz) for the GPS C/A-code signal, while the mid-range receiver uses a much wider band. The latter translates into capabilities for multipath mitigation by receiver signal processing (hence, smaller biases compared to the low-end receiver). In turn, that translates into higher measurement precision (smaller standard deviation values) and together in clearly smaller 95-percent error figures.
Again, we want to point out that these SF-PPP results are truly kinematic; they include no modeling of vehicle dynamics whatsoever. The measurements are processed recursively, epoch-after-epoch. Receiver position coordinates are estimated together with the receiver clock error, each epoch anew, and only the carrier phase ambiguities are propagated — as constants — from one epoch to the next (as long as no cycle slips occur).
Conclusions
A 95-percent position error of less than 1.75 meter is met in all cases for the horizontal coordinates. As a lane on a motorway in the Netherlands is 3.50 meters wide, these results show that real-time SF-PPP can be used for lane identification, when relying on fairly inexpensive equipment. The kinematic test showed a SF-PPP solution availability of larger than 99 percent.
Acknowledgments
The continued provision of RETICLE products by André Hauschild at DLR (German Aerospace Center) is acknowledged. The u-blox TIM LP receiver was kindly made available for the experiment by Mr. Sam Storm van Leeuwen.
Additional Resources
[1] Dow, J. M., and R. E. Neilan, and G. Gendt, “The International GPS Service (IGS): Celebrating the 10th Anniversary and Looking to the Next Decade,” Advances in Space Research 36, Vol. 36, No. 3. pp. 320–326, 2005
[2] Hauschild, A., and O. Montenbruck, “Real-time Clock Estimation for Precise Orbit Determination of LEO-Satellites,” Proceedings of ION GNSS 2008, pp. 581–589, Savannah, Georgia, USA, September 16–19, 2008
[3] Kouba, J., “A Guide to Using International GNSS Service (IGS) Products,” online publication at IGS website, 2009
[4] van Bree, R. J. P., and C. C. J. M. Tiberius (2011), Real Time Single Frequency Precise Point Positioning – accuracy assessment. GPS Solutions, DOI 10.1007/s10291-011-0228-6, 2011
[5] van Bree, R. J. P., and C. C. J. M. Tiberius and A. Hauschild (2009), “Real Time Satellite Clocks in Single Frequency Precise Point Positioning,” Proceedings of ION-GNSS-2009, pp. 2400–2414, Savannah, Georgia, USA, September 22-25, 2009