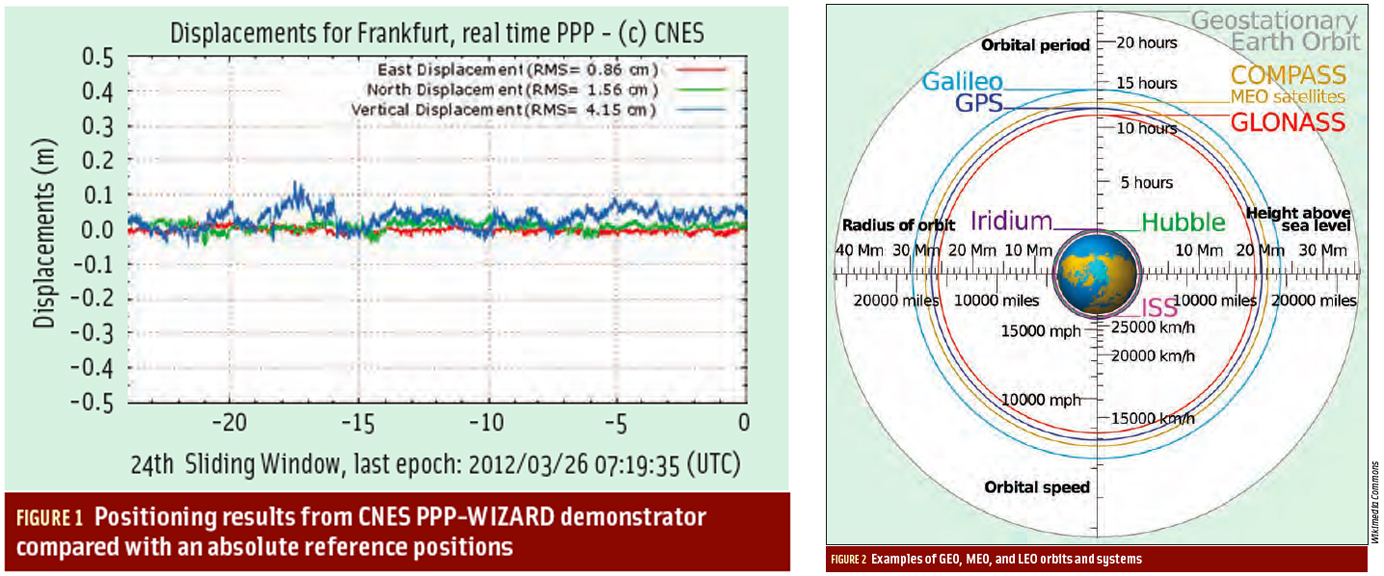
The navigation message has always been considered an inherent and essential feature of a global satellite navigation system. Its primary objective is to provide receivers with information on the errors of satellite clocks and parameters to compute the positions of satellites, as well as other parameters that help generate more accurate measurements.
The navigation message has always been considered an inherent and essential feature of a global satellite navigation system. Its primary objective is to provide receivers with information on the errors of satellite clocks and parameters to compute the positions of satellites, as well as other parameters that help generate more accurate measurements.
However, the architecture of the Global Positioning System, which has been copied — but not yet equaled) — by the other GNSSes, was conceived at a time when ranging was calculated on the basis of code measurements only and when satellites were rarely in connection with their ground segment.
Technologies have evolved since GPS’s inception, and GNSS architecture should evolve, too, in order to take into account the current reality: most receivers today are able to not only make code measurements but phase measurements as well. The latter are much more accurate, but also use much more computation power.
The expected evolution of GNSSes will enable receivers to achieve an accuracy on the order of a few centimeters, in real time, anytime and anywhere. The technology behind this evolution is already available and will improve traditional satellite navigation by two orders of magnitude (meaning that the accuracy will be about 100 times better).
This evolution is expected to be as significant in terms of improving accuracy and as important to society at large as the termination of Selective Availability for the Global Positioning System in 2000. As most will recall, Selective Availability was a function in the Global Positioning System that deliberately introduced random clock errors on the open signals used by civilian users to degrade those satellite signals, while special military receivers would be able to use the more accurate, encrypted P(Y)-code signals.
In this article, I will address the capabilities of GNSS precise point positioning (PPP) techniques and the need and possibility for GNSS operators to implement a global PPP capability.
An Insatiable Quest for More Accuracy
At the outset, we need to draw the attention of civilian users to the fact that the GPS open service (the so-called Standard Positioning Service or SPS) was not implemented as a separate service for civilians, but rather as an integral part of the military service (the so-called Precise Positioning Service, PPS). Until recently, all military receivers used the SPS C/Acode to synchronize their clocks and thus help them acquire and track the encrypted military signals (P/Y-code).
Thus the initial interest of the U.S. Department of Defense (DoD) in the SPS was not so much to provide a free public service to mankind. However, the constraints of cryptology and the state of electronic engineering at the time GPS was designed left it with no other choice than to provide an open service. Since then, of course, methods have been developed to enable military users to gain direct access to the P/Y-code without the need for SPS.
When the Global Positioning System began operation, however, SPS accuracy turned out to be much better than expected and was initially considered as a national security threat by the United States. Consequently, the DoD degraded its accuracy through the aforementioned Selective Availability until it realized, in the light of the experience of the first Gulf War and the war in Bosnia, that the drawbacks of the global degradation of an open service outweighed the expected tactical advantages. Furthermore, many civilian navigation experts had found ways to circumvent this voluntary degradation.
The termination of Selective Availability in May 2000 coincided with the take-off of the use of this service in mass-market products, initially for land vehicle navigation. This decision has certainly been instrumental in the widespread use of satellite navigation, as the pre-2000 GPS open service accuracy — while good enough for en route positioning of maritime vessels and aircraft — was inadequate for road navigation.
Since 2000, the Department of Defense has gradually improved the accuracy of its system, for both open and military services. Better SPS accuracy no longer seems to be perceived as a security threat. In any case, the Department of Defense always has the option to resort to local jamming in times of war or crisis to protect its assets, and those of its allies, against weapons and ordnance guided by satellite radionavigation.
Hence, whatever the original intentions of the operator of the Global Positioning System were, its open service has become the first truly global public service. Today, this system may have become the first operational military system in which civilian benefits to society outweigh its military benefits.
Historical Dependence on External Aids
Even though the DoD has pushed the accuracy of its open service to the limit in the current design of the system (now three to four meters for a single “standalone” receiver), this accuracy is considered not entirely satisfactory by many users.
GNSS accuracy is plagued by various types of errors linked to either the satellite system or the physical nature of the Earth (e.g., refraction and attenuation of signals propagating through the troposphere and the ionosphere). The sum of these errors can be easily measured at any point on Earth, if the actual coordinates of a receiver are known accurately, and are likely to remain valid in the vicinity of a given point over a small period of time.
Corrections provided by reference stations have greatly improved the accuracy of positioning since the advent of the Global Positioning System. This correction method is popularly known by the term “differential GPS,” but we will refer to it hereafter as differential augmentation.
A number of mostly public initiatives have emerged in order to offer real-time differential augmentation, which has proved to be a useful complement to the Standard Positioning Service. Some mapping agencies have developed differential augmentation services on a national scale, primarily through a network of terrestrial reference stations that broadcast corrections. Civil aviation authorities have developed continent-wide systems of differential augmentation based on measurements provided by a network of reference stations and correction data delivered via geostationary satellites:
- the Wide Area Augmentation System, developed by the Federal Aviation Authority of the United States and operational since 2005;
- the European Geostationary Navigation Overlay Service (EGNOS), developed by the European union and operational since March 2011 for civil aviation;
- the Multi-Functional Satellite Augmentation System (MSAS), set up by Japan and operational since September 2007.
Various commercial differential augmentation systems can achieve accuracy levels from a meter down to 10 centimeters or better. But such accuracy levels are still considered insufficient for a number of civilian applications, notably for surveying, some Earth observation data, and precise vehicle guidance for such things as off-shore exploration, mining vehicle operations, and automated farming.
In order to achieve better than 10-centimeter accuracy, satellite navigation must resort to the intricacies of the physics applicable to radio waves and the characteristics of signals in space. The accuracy of positioning is directly proportional to the accuracy of satellite-to-receiver ranging and also is very dependent on the accuracy of the computation of the position of the satellites and their clock errors.
The first mass-market GPS receivers were mostly only able to measure the codes of the signals and associated navigation messages. Today, receivers can usually process carrier phase measurements as well.
In order to calculate the unknowns or integer ambiguities linked to phase measurements, two primary approaches have been employed over the last 15 years:
- using differential augmentation through a reference station — a technique known as real time kinematic (RTK)
- obtaining new and better information on the position and clock error of satellites — known as precise point positioning (PPP).
- Both approaches have pros and cons, which have been extensively described in the professional literature, and we will briefly summarized them here. (See also Additional Resources.)
- RTK delivers an instantaneous high-accuracy solution, in the static and dynamic modes, but only in the vicinity of a reference station, the position of which is precisely known. This technique implies a communication channel from the reference station to the receiver and has led to a number of commercial services offered by private companies such as NavCom Technology, OmniSTAR, and Veripos.
- PPP was initially used in post-processing mode, that is to say a posteriori, by a server which receives the observables made by the moving receiver for a specific fix and which has privileged information on the exact position and clock errors of satellites. Some public organizations recently made available such information in quasi–realtime on Internet websites so that the post-processing computation can also be made in real-time. The barrier to the success of such websites remains the availability of such information for the vast majority of receivers, which do not have access to the Internet. Real-time augmentation, as opposed to the post-processing augmentation, has proved feasible through the broadcast of the exact orbit (ephemeris) and clock error of the navigation satellites through geostationary satellites. Unlike RTK, PPP is operational worldwide and does not require a reference station. One of its drawbacks, however, is that the real-time augmentation requires a warm-up time (also called the convergence time) of about 30 minutes for receivers.
The two techniques could be summarized in the following very simplistic manner (where “precise location” means the accurate location inferred from code and phase measurements, which is much more accurate than the location inferred from code measurements only):
- RTK: “Tell me the precise location of your reference station and I will tell you the precise location of your mobile receiver.”
- PPP: ”Tell me the precise location of the navigation satellites and I will tell you the precise location of your mobile receiver.”
The main drawback of precise point positioning, namely the convergence time for receivers, can be mitigated by the following actions:
- The natural increase in computation power of chipsets (under Moore’s law) will help decrease the convergence time in the future.
- The use of several constellations for PPP was proved to decrease the convergence time (See the article by C. Cai and Y. Gao in Additional Resources).
- The use of more than two frequencies could also help decrease the convergence time.
The two aforementioned techniques have been further developed with different variants over time. For example, RTK uses a network of several reference stations instead of only one. PPP has been employed based on either one, two, or three navigation frequencies, and/or one or more constellations, including a modeling of satellite biases (See the article by D. Laurichesse et alia).
However, both techniques have something in common: They both remedy the incapacity of the navigation messages of the current global navigation satellite systems to enable receivers to benefit from phase measurements. The architecture of navigation messages is designed to give valid parameters on orbits and clock corrections over long periods (typically one to three hours). This is appropriate and accurate enough to achieve positioning through code measurements only but not enough to benefit from phase measurements.
Both techniques seem to offer equivalent accuracies. In addition, they are also not mutually exclusive and can be combined. However, only PPP can offer a global and standalone augmentation solution (i.e., without the support of a reference station). This is why this technique is expected to become the next step in satellite navigation.
Channel Options for PPP Correction Data
The question that should come to mind for all users is: Why can’t navigation signals give me the requested highly accurate information on the position of satellites and clock errors so that I can perform accurate positioning using phase measurements?
The reason for the current situation is twofold. First, the ground infrastructures of GNSS systems have not been designed to support a continuous flow of correction data to the satellites; instead, the corrections are uploaded intermittently. In any case, operators of these systems do not directly provide the real-time ephemerides and clock corrections of satellites. This is not because the operators are technically unable to perform such calculations; rather, the need has not yet presented itself nor have the service providers been mandated to do so.
Fortunately, other organizations, such as the International GNSS Service (IGS), with a larger number of tracking stations do produce this information. The French Space Agency, CNES, has developed a project, the PPP-WIZARD demonstrator, as a “proof of concept” of the zero-difference ambiguity resolution method based on the agency’s orbit determination service.
Figure 1 (see inset photo, above right) shows an example of PPP results compared with an absolute reference position using CNES’s software, in real time, and incorporating a complete set of correction data from GPS and GLONASS satellites. In this case, the data comes from the Reykjavik, Iceland, reference station of the EUREF Permanent Network, which also contributes data to the IGS.
The peculiarity of the current situation is that correction data enabling PPP are available for free on the Internet but available over space links only for a limited number of high-end users able to pay fees for a worldwide service, requiring payloads on approximately 10 geostationary satellites.
The viewpoint of the author is that the correction data enabling PPP should become an integral part of the data messages of all GNSS signals, ideally for free, since those correction data have become de facto public data. This position is based on the following reasoning.
Are there better ways to send correction data to receivers?
Terrestrial broadcasting is obviously not the best solution because it would be dependent on initiatives taken at national or local levels, resulting in different standards and/or transmissions on different radio frequencies. Moreover, this approach would imply additional connection fees and, potentially, roaming fees each time a user navigates outside the national borders of his or her telecommunication provider.
The Internet, through terrestrial radio broadcasting, poses the same issue of connection and roaming fees as well as potential latency issues inherent in use of the Internet. This is why satellite broadcasting should be preferred over terrestrial broadcasting.
The choice of satellite broadcasting theoretically gives rise to three options: using low Earth orbit satellites (LEOs), medium Earth orbit satellites (MEOs), or geostationary satellites (GEOs). (See Figure 2, inset photo, above right.)
The best candidates amongst current LEOs are telecommunication constellations (such as Iridium or Globalstar). However, the broadcast of correction data through such satellites would consume a significant chunk of data bandwidth on two-way communication channels, probably requiring huge connection costs, not to speak of the necessity of equipping receivers with a satellite phone terminal.
In any case, for practical purposes, GEOs are better placed than LEOs for one-way communication. So, the real choice in correction data channels should be between MEOs (only navigation satellites up to now) and GEOs.
Broadcasting from a constellation of MEOs offers significant advantages compared to geostationary satellites:
- better coverage of natural and urban canyons and of polar latitudes;
- reduced risk of accidental masking and its consequences (potentially the need to reinitiate the convergence period for receivers);
- no need for dedicated broadcast payloads (and associated connection fees) on GEOs, since payloads on navigation satellites already exist.
In addition, as the only satellites in MEO orbits so far are satellites of the four existing GNSSes, correction data could well be broadcast on their respective navigation signals. This would have the very desirable benefit of enabling receivers to monitor one fewer radio frequencies since they are already tracking those signals anyway.
In turn, that would simplify receiver design and almost certainly lower power consumption of user equipment. It would also enable PPP correction data to become an inherent service of GNSSes.
Technical and Political Obstacles
PPP delivered by a global navigation satellite system still faces technical challenges to overcome before becoming a reality:
- Continuity over time of the connectivity from uplink stations to satellites is not ensured today with the design of the current GNSSes. However, this could be solved through more uplink stations, more antennae at these stations, and/or the introduction of inter-satellite communication capacities.
- The information must be multiplexed in a clever way in order to mitigate the effects of handovers, where a satellite switches from one station to another, and of incidental masking of satellites.
However, the main hurdle to the advent of PPP delivered via GNSS is likely to be of a political nature. Indeed, why should the operators of such systems invest in the necessary upgrades to the architecture of their current ground segment?
Decision-makers will be primarily concerned with the questions of whether the evolution toward built-in PPP will bring benefits to governmental users in the first place and whether it constitutes a boon only to existing high-accuracy users, who already pay for similar high-accuracy services.
Would PPP benefit governmental users, including military users? The answer is “yes.” High accuracy navigation could enable helicopters, jets, and drones to fly close to the ground with little or no visibility even in difficult environments (such as mountainous and rocky areas). It will also benefit a number of civilian governmental users: police forces, agriculture agencies, geodetic survey and mapping agencies, and so on.
The potential contribution of PPP to civil aviation has not yet been extensively studied, but we can imagine that, in the future, global PPP would offer a high level of safety and even compete with existing publicly operated satellite-based augmentation systems (SBASes) currently used by civil aircraft (see the article by J. Booth and R. Snow in Additional Resources).
Does that mean existing private service providers of high-accuracy services should fear the advent of global PPP? The answer is “no.” Commercial service providers have started to offer high-accuracy solutions as a marginal service, that is, as an add-on to their core business in order to satisfy the needs of their professional clients. If these companies have engaged in remedying the limitations of the Global Positioning System, they have never had the need or capability to supplant it — indeed, their business model is based on adding value to the basic resource provided by GNSS systems.
Moreover, the added value does not lie in the broadcasting of navigation parameters but in the intelligence of the software used for automating mining vehicles, guiding high accuracy farming tractors, piloting off-shore vessels, and so forth. Global PPP would offer service providers the opportunity to focus on segments with the most added-value and enable them to develop new services using the enhanced GNSS capabilities to offer to their clients. It would not decrease, but rather increase their commercial opportunities.
High Accuracy: Technology Push
If, or when, a GNSS system operator announces its decision to broadcast correction data enabling PPP and publishes the interface document of this new service, this shift is likely to trigger the development of new generations of receivers amongst manufacturers, new services for applications requiring high accuracy, and new digital maps with much higher resolutions.
This shift will not constitute a revolution, as was the case with the termination of Selective Availability in 2000. More likely it will be a long evolution, where the advantages progressively materialize as the ground segment is complemented stepwise. This high accuracy is very likely to become addictive to the point of becoming the new standard for mass-market receivers.
Such an evolution towards PPP on GNSSes may also become a formidable opportunity to foster more international cooperation amongst system operators, notwithstanding the defense dimension of such systems. Indeed, not only time offset and observables should be exchanged, but correction data should also be standardized amongst systems in order to make the life of users easier. Such cooperation would truly enable real-time interoperable PPP positioning using all available GNSS constellations and frequencies.
Given that the PPP technique can no longer be ignored, deciding not to deliver correction data enabling this type of augmentation would constitute a “Selected Unavailability.” In other words, it would represent a deliberate decision by system operators not to deliver over their satellites a commodity that is already available on the ground: the correction data needed by PPP-capable user equipment.
To conclude, convincing arguments exist to expect that GNSSes will commit to PPP as a complement to traditional satellite navigation. The question is not whether they will agree to this, but rather when, and which system will move first.
Author’s note: The views expressed in this article are personal and do not reflect the opinion or position of the European Commission.
Additional Resources
[1] Bisnath, S., and Y. Gao, “Innovation: Precise Point Positioning,” GPS World, April 2009
[2] Booth, J., and R. Snow, “Help from Above, Flight Testing Two Alternatives to Ground-Based DGPS,” Inside GNSS, pp. 26-35, September 2010
[3] Cai, C., and Y. Gao, “Precise Point Positioning Using Combined GPS and GLONASS Observations,” Department of Geomatics Engineering, University of Calgary, Alberta, Canada, Journal of Global Positioning Systems, Vol.6, No.1: 13-22, 2007
[4] French Space Agency, see here
[5] Fugro
[6] German Mapping Agency, Bundesamt für Kartographie und Geodäsie, see here
[7] Jet Propulsion Laboratory of the National Aeronautics and Space Administration, see here
[8] Kostadinov, A., and V. Broederbauer, and R. Weber, Real time Precise Point Positioning, Vienna University of Technology, Geophysical Research Abstracts, Vol. 7, 06630, SRef-ID: 1607-7962/gra/EGU05-A-06630, 2005
[9] Kouba, J., A Guide to Using International GNSS Service (IGS) Products, May 2009
[10] Laurichesse, D., and F. Mercier, J. P. Berthias, P. Broca, and L. Cerri, “Integer Ambiguity Resolution on Undifferenced BPS Phase Measurements and its Application to PPP and Satellite Precise Orbit Determination,” NAVIGATION (Journal of the Institute of Navigation), Vol. 56, No. 2, Summer 2009
[11] Mireault, Y., and P. Tetrault, F. Lahaye, P. Heroux, and J. Kouba, “Online Precise Point Positioning” GPS World, Vol. 19, No. 9 (September 2008), pp. 59–64
[12] Natural Resources Canada, Canadian Spatial Reference System