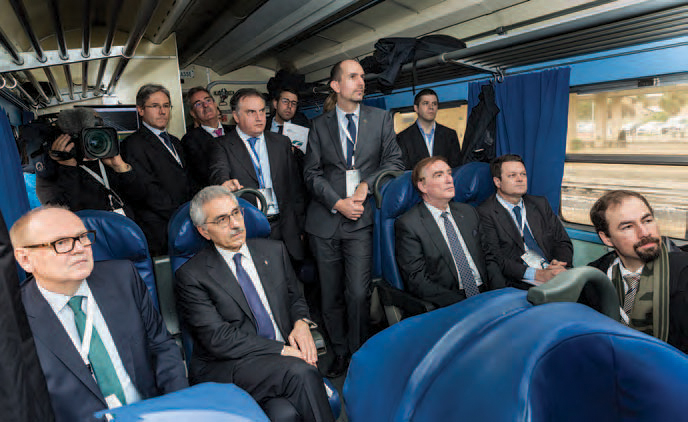
The train control segment remains one of the most important components of the railway market today, accounting for €12.7 billion, or about $13.5 billion dollars, out of the world rail market, which amounts to about €150 billion (about $159.3 billion), according to the latest studies by UNIFE (The Association of the European Rail Industry). In the railway domain, this control segment also is one of the most important in terms of industrial and technological innovation, in particular in Europe with the development and the continuous improvement of the European Rail Traffic Management System/European Train Control System (ERTMS/ETCS) standards.
In the last two decades the ERTMS/ETCS has been developed by the European Union (EU) to not only enhance safety and increase efficiency, but more importantly to enhance cross-border interoperability and foster the development of a unique European rail market that is characterized by a high number of very different legacy systems in each country working with widely different regulations.
Early Objectives
The first aim of the development of the market pillar. It didn’t prohibit the possibility of developing a high-quality standard for the train control system that could be implemented all over the world. At the end of 2016, some 90,000 kilometers of ERTMS lines have been contracted in the world with about 12,000 vehicles representing a de-facto ERTMS/ETCS standard was, then, focused on the single standard and a laudable benchmark in terms of safety and interoperability. Figure 1 shows the architecture of the ERTMS Level 2, the dominant solution being promoted today.
The European regulations called for an implementation and migration plan for the new standard requiring that all the core TEN-T network be equipped with the ERTMS/ETCS technology by 2030, and the entire comprehensive TEN-T network by 2050. It also provides that no significant improvement be implemented on the existing legacy systems, in order to further incentivize the transitions from these systems to the new European standard.
Some European countries, going beyond the European regulations, have already decided on a full and accelerated transition from their own legacy systems to the new standards on the whole national networks. This is the case in Belgium, Denmark, Luxembourg, Sweden, Switzerland and Norway (these two last countries decided for a network-wide implementation of ERTMS/ETCS even while not belonging to the EU).
Due to the high quality of the European system, ERTMS/ETCS specifications are becoming the standards for most of the world train control systems, even those outside of Europe. In some cases the system was chosen as developed by the EU, while in other cases the ERTMS/ETCS specifications have been adapted to the local framework. A network-wide development of the ERTMS/ETCS technology has been decided by the authorities of some countries outside Europe, including Algeria, Libya, Australia, China, India, South Korea, Saudi Arabia, and Taiwan.
A study performed by Bocconi University (Milan, Italy)estimates that the ERTMS/ETCS technology potential market could rise up to a quarter of the world market by 2030 and to half of the world market by 2050.
Despite the great success of the ERTMS/ETCS specifications, the system does have its limitations, in particular in terms of cost or economical sustainability especially for its deployment on local, regional and low traffic lines.
The ERTMS (European Rail Traffic Management System) consists of two main components: the ETCS (European Train Control System), that is a standard for in-cab train control, and the GSM-R, the GSM mobile communications standard for railway operations, that requires a dedicated telecom network whose costs range between €40,000-60,000 per kilometer (about $42,140-63,658) and whose technology is reaching the obsolescence.
An interesting alternative to GSM-R is represented by the so-called IP-based bearer independency accepting the use of public networks instead of dedicated networks. Another innovation proclaimed since the early stages of ETCS’s conception — but not given serious consideration until recent years — is the use of GNSS to localize the train. Both of these components are now being studied around the globe by the train control industry in order to decrease costs and foster the system deployment through innovations. For more details, see the Additional Resources section near the end of this article.
New technology “game-changers” such as IP-based telecom bearers and satellite localization were introduced at an ERTMS stakeholders meeting at the Innotrans fair in Berlin (September 20, 2016) where the new ERTMS Memorandum of Understanding (MoU) was signed (Figure 2).
Current ETCS specifications require that the train is localized by its odometer whose error is reset intermittently through balises, georeferenced points located along the line. The innovation consists of introducing the concept of a virtual balise that would be functionally equivalent to the physical balise, an electronic beacon deployed along a railway track at a georeferenced point (See ). This method prevents changing the current specification and the system architecture proposing a continuous localization system through satellite, and then assuring interoperability between current technologies. Figure 3
The detection of the virtual balise would be performed by an on-board virtual balise reader providing the absolute train location to the train control system, while the odometric subsystem measures train speed and distance travelled, then provides a relative positioning with respect to the last detected physical balise.
The main advantage of this technological innovation consists in the savings related to the not-installed physical balises and to the related maintenance that could become increasingly costly for the infrastructure manager, particularly on those lines that are not easily accessible. The need for providing a specific on-board virtual balise reader does represent an increased cost that has to be valued against the potential savings of the ground side.
Reference Platform
As a reference, the ERTMS platform is designed to operate with virtual balises — tasked with the same function of physical balises for the train localization — and uses public GSM/LTE/Satellite networks instead of the GSM-R dedicated network. Studies and research activities are being developed to assess the autonomy of the virtual balise concept and to identify operational conditions under which the virtual balise concept can replace the deployment of physical balises along the lines.
This is the case of railway lines with long tunnels or with a high complexity in terms of forks and junctions, that may require the presence of some physical balise in complement to the use of the GNSS localization.
Furthermore, the generation of virtual balises implies a proper interface with a GNSS Augmentation network and the ERTMS system to over bound the positioning error according to the safety requirements of ERTMS.
Figure 4 shows the architecture studied to implement the virtual balise functionality in Figure 3. The interface with the ERTMS Radio Block Centre (RBC) is the Track Area Local Server (TALS) introduced for processing the augmentation messages supplied by either dedicated and/or public augmentation networks including European Geostationary Navigation Overlay Service (EGNOS) and Wide Area Augmentation Sysztem (WAAS). The augmentation messages, after being processed by the TALS, are transmitted to the GNSS receivers of the trains through the secure telecommunication link of the ERTMS system. Multiple constellations (i.e., GPS + Galileo) and other localization means based on telecom signal of opportunities can enhance the availability and resiliency of train localization.
The train localization is provided by the virtual balise reader by processing the GNSS signals of two independent receiver chains with a track data base describing the geometry of the rail segments. Furthermore, coherence checks (independently from GNSS) are provided by the ERTMS diagnostic means to achieve the high Tolerable Hazards Rate (THR = 0.5 * 10-9 / hour) required by the ERTMS, which otherwise could have not been provided by the receiver itself. When the train passes on a point where a virtual balise is located, it identifies the location and transmits it to the RBC. The RBC then compiles information from the interlocking and trains in its control area and sends movement authorities and other information to individual trains, taking into account a safe distance to the train ahead.
On this basis, the train on-board unit (OBU) is able to calculate its braking distance and optimal speed. The train’s OBU is composed by the ETCS standard modules, a new TLC module able to transmit and receive GSM-R, GSM, LTE and satcom signals, and a physical balise reader in case some physical balise is needed on the ground side to ensure interoperability on other lines equipped with the standard ERTMS solution.
Economical Analyses
An impact analysis aims to evaluate the conditions of economic conveniences from the introduction of a new satellite technologies solution for the evolution of the ERTMS system. A quantitative analysis has been carried out within the ERSAT EAV project based on the Cost-Benefit Analysis (CBA) methodology and focused on regional and local lines in Europe. The total market in Europe has been conservatively estimated to be 28,000 line kilometers where different legacy systems are installed, many outdated and in several cases still manually operated. The goal is to replace these older systems with the ERTMS based on GNSS and multi-bearer telecom providing the new investments are economically sustainable.
The CBA methodological framework took into consideration the following cost elements provided by RFI and DB Netz, the railways Infrastructure Managers (IM) in Italy and Germany, and by Ansaldo STS, which is delivering the first GNSS-based train control system in Australia:
- the economic effects are assessed at the system level, from the society’s point of view;
- the economic effects of the project scenario are evaluated in differential terms with respect to an alternative scenario, which is the upgrade to the traditional ERTMS;
- the analysis is applied considering a time horizon defined taking into account the life cycle of the main investment and it uses a discounted flows technique.
Under these circumstances the CBA shows that the potential benefits far outweigh the costs of introducing a GNSS-based ERTMS with advantages depending on the status and complexity of the single line. Since cost savings are due to the reduction of physical balises and telecom infrastructures, a proper sensitivity analysis has been made to evaluate the impact of maintenance costs of balises and the availability of GSM-R infrastructures (Figures 5-6).
Ground equipment costs slightly more for IMs (the owner and operators of the railways network) because of additional components in the RBC (namely the TALS and the track database). For Railways Undertaking (RUs) — the train owners that provide the service to passengers, the required investment in the OBU is also higher in the project scenario — the layout of OBU in the project scenario includes: standard modules, (including ETCS), a VBR (Virtual Balise Reader), a BTM (standard Balise Transmission Module since physical balises are still needed in some critical points of the network – e.g., level crossings), and a MAR (Multipath Access Router) which selects the public telecom bearer including the GSM-R.
The main benefits for IMs derive from the reduced need for physical balises, which translates into savings during both the investment and the operating phase.
Collateral benefits also can be assessed for RUs due to the possibility of optimizing the train driving, reducing both the time spent at the start of a mission (when the train needs to meet a physical balise for its position to be safely assessed) and the events of unnecessary brakings and slowdowns deriving from failures in the balise-BTM communication.
However, in the project scenario, the accuracy and availability requirements are assumed to generate (at least in the first part of the time horizon) the need for a further level of augmentation of the satellite signal being provided by a satellite-based augmentation system (SBAS), which represents an additional cost for IMs.
In terms of the telecommunication part, the project scenario benefits from removing the need to invest in GSM-R while making use of already available public/commercial infrastructure and more affordable services.
All in all, the GNSS-based solution proves to be especially convenient because of relevant savings in both capital and operating expenses, with final Benefit/Cost Ratios well above 1 in all case studies examined.
However, most of these savings are captured by the IMs. The business case for RUs is a more complex issue since both VBR and BTM are necessary to ensure a train operates with traditional balises and virtual balises as well. Nevertheless, in new green field lines it is possible to eliminate the BTM, thus reducing the investment for RUs. The defined scenarios do not look ahead to impacts which directly benefit the balance sheets of RUs, but some indirect “collateral” benefits have been identified. Some of these benefits have been estimated within the CBA, while others cannot be estimated quantitatively because they depend on several factors linked to the policy and strategies of other players, but are worth mentioning.
Additionally, some of the gains that can be highlighted also affect the convenience of the project at the system level and are capable to lead it to higher Benefit/Cost Ratios.
• TLC costs and infrastructure charges. Currently the fee for TLC services used by RUs is generally included in the infrastructure charges paid by RUs to IMs, as IMs are the providers of GSM-R services. In the project scenario, the value chain of the telecommunication services would change, and the prudential assumption applied in the CBA was that it would be the RUs to pay direct fees for TLC service to the TLC and satcom provider. However, this means that the project scenario includes operating TLC costs for RUs that the baseline scenarios does not consider – since no assumption on a corresponding decrease of infrastructure charges was adopted. As a matter of fact, the decrease of infrastructure charges as a way to partially transfer the benefits of the GNSS-based scenario from IMs to RUs is a possibility that – while difficult to estimate – has to be taken into account as a favorable option for the railway system as a whole. Even if this was limited to the amount of TLC costs (so that the shift from the baseline scenario to the project scenario was cost-neutral for RUs in terms of telecommunications), the benefits for the business case of RUs would be relevant, putting their Benefit/Cost Ratio at 1.14.
• Additional costs of OBUs and Virtualization of all balises. The main disadvantage for RUs, and the main item which makes their business case weak is the need to upgrade the OBU at a higher cost than the ERTMS scenario would imply. In fact, there are three sensitive assumptions in the project scenario: (1) the OBU will still include a BTM. This derives from the fact that physical balises are still necessary today for allowing accurate positioning of the train in certain critical points of the network; (2) the unit cost of MAR; and (3) the unit cost of VBR. The combination of these assumptions implies an additional cost that can increase the cost of OBUs of up to 16%. By reducing the additional cost of OBUs in the project scenario (compared to the baseline scenario) it would be possible to further contribute to the business case of RUs, moving their BCR above 1.32 in the theoretical case where OBUs for the GNSS-based ERTMS would not cost more than in the traditional ERTMS scenario.
At the system level, this solution is beneficial even under prudential assumptions. However, a major issue is avoiding the need for an additional ground-based augmentation network and equipment by improving the performance of satellite-based augmentation combined with existing ground network.
The basic rationale of introducing satellite technologies in the rail sector is to generate economic benefits and spread them among the users by simplifying the infrastructure and equipment needed. As indicated by the economic analyses, minimizing the need and/or the impact of additional “hard” elements, as compared to alternative solutions, is of utmost importance to exploit the benefits (given by the virtual balise concept) and make this solution ground-breaking. To this aim, the RHINOS (Railway High Integrity Navigation Overlay System) project coordinated by Radiolabs and involving GPS experts at Stanford University, DLR, University of Nottingam and University of Pardubice is studying a way to define the protection level to comply with the challenging requirements of the ERTMS safety standards. For more details, see the Additional Resources section. The ultimate goal is to re-use existing and future GNSS augmentation systems world-wide in a seamless fashion as is the case in the aviation sector.
Operational Aspects
The ERTMS system guarantees a safe train front end by using its odometry to measure the distance from the previous valid balise (last relevant balise group). The error accumulated by the odometry (confidence interval) is reset once a new balise group is encountered. Looking to Figure 7, the confidence interval that must be guaranteed within a THR of 0.5.E-9/hour, is bounded by the virtual balise location accuracy plus the over/under reading amount of the odometer that is a function of the travelled distance.
The magnitude of the confidence error is taken into account to ensure a safe distance with the preceding train. However, the most stringent train positioning requirement is in the order of three meters to dis-criminate the track where the train is located. The positioning of virtual balises is a matter of trade-off between the surrounding environment to limit the multipath effects, and operational constraints. The multipath represents the most important threat for rail application and to this respect the characterization of possible operational scenarios is an important issue under investigation in the STARS project. With respect to physical balise whose error is one meter, the virtual balise error is probabilistic (Figure 8) and must be computed, assessed and validated in the rail environment.
From an operational point of view, the introduction of virtual balises and the use of public telecom networks represents a step change innovation for ERTMS. In fact, these technologies belong to other entities not strictly under the control of the signalling suppliers and infrastructure managers. Nevertheless, a safety case must be produced including these technologies, and specific interfaces are mandatory to define a Service Level Agreement (SLA) with the providers of augmentation services and telecom services. Figure 9 shows the relationship among different players.
These providers are not yet in the field as is the case in the aviation sector where the Air Traffic Control agencies have created European Satellite Service Provider (ESSP) as a service provider for EGNOS.
In the United States, where the Federal Railroad Administration continues to support railroads in implementing Positive Train Control (PTC) — a processor-based/communication-based train control system designed to prevent train accidents — telecom services are being provided by specific companies to federate the demand and reach economy of scale, while in Australia, the Australian Rail Track Corporation (ARTC) has involved major telecom operator Telstra, which uses both cellular and satellite technologies, to provide telecommunications to the national rail freight network. ERTMS has a fundamental requirement of interoperability, and until now the GSM-R standard has guaranteed this requirement, leaving competition at the supplier level. With the new technologies a shift from capex to service is inevitable to capture the economy of scale. Service providers will likely be part of the signalling integrator supply chain and must guarantee interoperability, cost effectiveness and technology innovation in order to overcome obsolescence of their products.
Important contributions to innovation on ERTMS are part of Shift2Rail — the first European rail joint technology initiative to seek focused research and innovation (R&I) and market-driven solutions by accelerating the integration of new and advanced technologies into innovative rail product solutions.
Conclusion
Exciting new capabilities and business models are making GNSS use a key contributor to deploying ERTMS on local and regional lines now and in the coming years. ERTMS has been successful in becoming the European train control system and in ensuring interoperability, competitivity, and export on international markets. GNSS use for train localization and public telecom networks are expected to produce significant benefits for the operational costs that are key to making ERTMS economically sustainable for both local and regional lines that represent the bulk of lines. Most of these lines are old and manually operated, and require huge investments in the next years.
A new service-based business model is emerging to fully exploit the benefits of these innovations and to allow the rail industry to provide turn-key systems. The results of the projects launched in 2012 are contributing to consolidate the roadmap and to create a consensus among the rail community. A remark-able synergy potential is expected by exploiting Galileo as a complement to GPS and other constellations, that together with ERTMS represent the two pilasters of the European industrial policy. As we look ahead, with new GNSS features coming on the field and increasing ERTMS needs, GNSS and ERTMS are becoming more tightly intertwined, amplifying their combined business models.
Additional Resources
1. 3InSat Project
2. ERSAT (ERtms on SATellite)
3. First RHINOS Workshop at University of Stanford marked bold commitment on high integrity GNSS for ERTMS
4. Marais, J., IFSTTAR “Etat des lieux des initiatives GNSS – Rail, JOURNÉE INNOVATION GNSS RAILTOULOUSE 24 MARS 2016
5. Next Generation Train Control (NGTC)
6. Railway Stakeholders Commit to Disciplined ERTMS Deployment
7. Shift2Rail Joint Undertaking
8. World’s Rail Market Study, Forecast 2016 – 2021, UNIFE
9. STARS – Satellite Technology For Advanced Railway Signalling