Identifying GNSS interference based on ADS-B Out data and validating that data against confirmed interference from fielded airborne receivers.
JEREMY KAZMIERCZAK, ANGELO JOSEPH, GEORGE COOK, COLLINS AEROSPACE
To fly within controlled airspace in the United States, aircraft must be equipped with an ADS-B Out transponder to broadcast aircraft identification information, position, velocity and time (PVT) information, as well as other performance and integrity indicators.
Air Traffic Control (ATC) uses this information to direct the flow of air traffic and maintain aircraft separation. Aircraft equipped with ADS-B In receiving equipment can use the broadcast for situational awareness and traffic avoidance. Any user with an ADS-B In receiver can use the ADS-B Out broadcast for aircraft identification and position.
The FAA has mandated the use of ADS-B Out for aircraft flying within U.S. controlled airspace since January 1, 2020, as part of its Next Generation Air Transportation System (NextGen) infrastructure enhancements [1]. Many other countries have adopted or are in the process of upgrading their ground infrastructure to use ADS-B Out information for similar ATC purposes.
There are now enough ADS-B Out equipped aircraft operating in the Continental United States (CONUS) to identify trends such as geographical location, altitude, time/date, aircraft fleet and when interference is encountered. This article discusses methods used to identify GNSS interference based on ADS-B Out data and validates those methods against confirmed interference from fielded Collins Aerospace airborne receivers. Tools were also developed to process the large amount of ADS-B Out data available and to display interference events in a useful manner to identify trends.
One primary sensor integral to the ADS-B Out function is the positioning source used to provide accurate and reliable PVT information to the transponder. Advisory Circular (AC) 20-165A [2], Appendix 2, defines the minimum performance requirements for an ADS-B Out positioning source. The positioning source may be external to the transponder or integrated as part of the transponder. It’s typically a Global Navigation Satellite System (GNSS) receiver or a tightly-coupled Global Navigation Satellite System/Inertial Reference System (GNSS/IRS).
For this reason, ADS-B Out functionality is highly dependent on GNSS availability for operation (for example installations using a GNSS receiver) as the positioning source may lose the ADS-B Out function as a result of losing a GNSS navigation solution.
A complete list of FAA approved ADS-B Out installations, including positioning sources, is available through the FAA’s website [3]. At the time of publication, the FAA ADS-B equipage website indicates more than 150,000 U.S. civil aircraft are equipped with ADS-B Out installations.
The use of ADS-B Out has grown globally, and so to has the availability of live and historical flight tracking services using ADS-B Out position. FlightAware, FlightRadar24, ADS-B Exchange and the OpenSky Network are all examples of public flight tracking services that provide ADS-B Out aircraft position reports. Previous publications have shown RF interference can be observed in ADS-B Out transmissions [4].
This article analyzes nine months of historical ADS-B Out data from FlightAware over the CONUS to map potential RF interference as it relates to airborne GNSS receivers used as ADS-B Out positioning sources. Reference [4] also lists isolated cases of confirmed Aviation GNSS RF interference events around the world. This article focuses on the CONUS for the following reasons:
1. The large number of ADS-B Out equipped aircraft operating daily in the region since January 2020.
2. The nearly 100% ADS-B receiver coverage over the CONUS.
3. The expectation that airborne GNSS interference should be minimal in the CONUS (as opposed to other regions around the globe where interference may be expected).
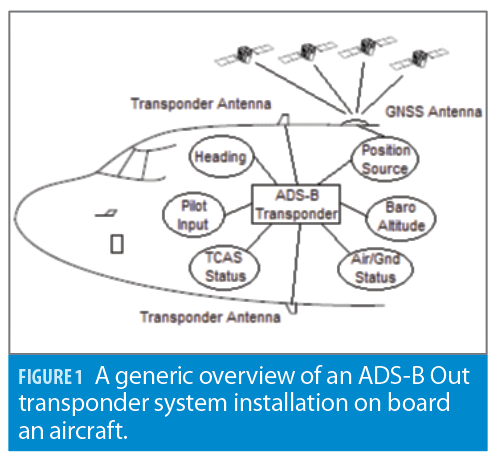
Confirming Airborne GNSS RF Interference
Figure 2 shows the Collins Aerospace GPS-4000S (-1xx) receiver, which is a TSO C145d [5] Class Beta-3 receiver and an FAA approved positioning source used in multiple turbine aircraft ADS-B Out installations. The GPS-4000S (-1xx) is fielded on multiple business jet and turbine engine sized aircraft platforms.
Figure 3 shows the Collins Aerospace GLU-2100 Multi-Mode Receiver (MMR), which is a TSO C145e [6] Class Beta-3, TSO C146e [7] Class Delta-4 and TSO C161a [8] receiver in addition to an FAA approved ADS-B Out positioning source used in multiple transport category aircraft installations. The GLU-2100 is fielded on multiple commercial passenger aircraft platforms.
Both the GPS-4000S (-1xx) and GLU-2100 receivers implement a simple RF Automatic Gain Control (AGC) based interference detector that is very effective in detecting narrow band RF interference by using the distribution of the A-D (Analog to Digital) bits. Both receivers log this A-D information as a method to confirm RF interference in the case of a loss of navigation during operation. Figure 4 shows a recorded epoch of the GPS-4000S (-1xx) receiver when there is no RF interference present. The A-D Bin distribution outlined in red shows a Gaussian signal representative of noise. The receiver is tracking 10 GPS satellites and three SBAS satellites outlined in red at the bottom of the figure.
Figure 5 shows the same information at an epoch seven seconds later when RF interference is encountered. At this time, the A-D bin distribution is inverted, or non-Gaussian, and the receiver lost track of four GPS satellites and two SBAS satellites due to the in-band RF interference. This A-D bin distribution behavior gets logged as an indicator of RF interference on both GPS-4000S (-1xx) and GLU-2100 products.
This behavior has been confirmed on both GPS-4000S (-1xx) and GLU-2100 equipped aircraft when the aircraft experienced a loss of GNSS or loss of ADS-B Out functions. Some of the RF interference events logged have occurred in regions of the world where RF interference is well documented and expected, and some events have occurred at locations where RF interference might not be expected. The purpose of this paper is not to identify interference sources, but rather to map when and where RF interference occurs to understand the scope or impact of RF interference to airborne receivers.
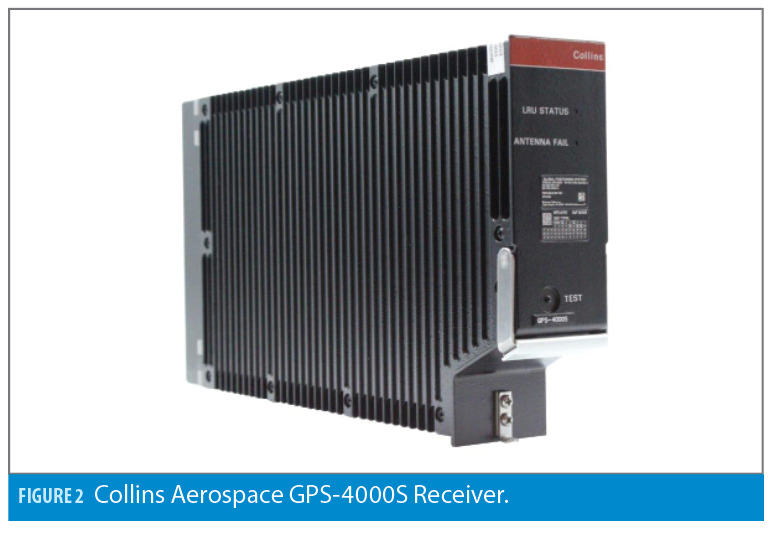
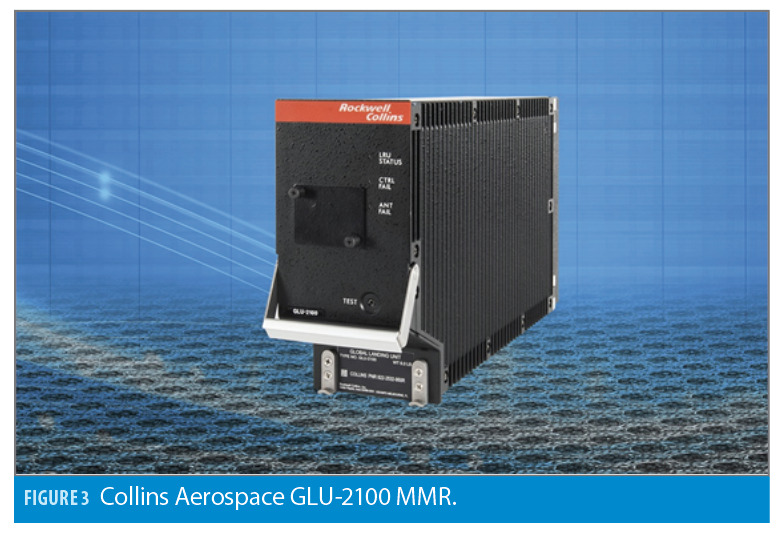
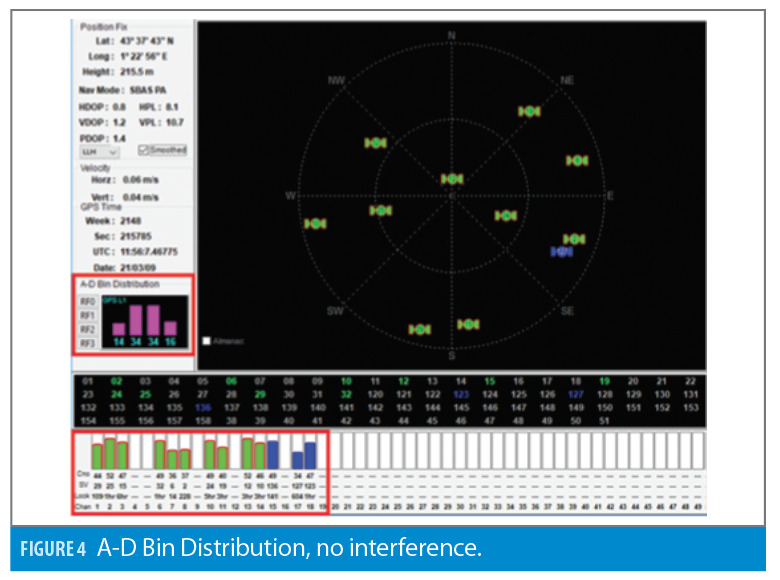
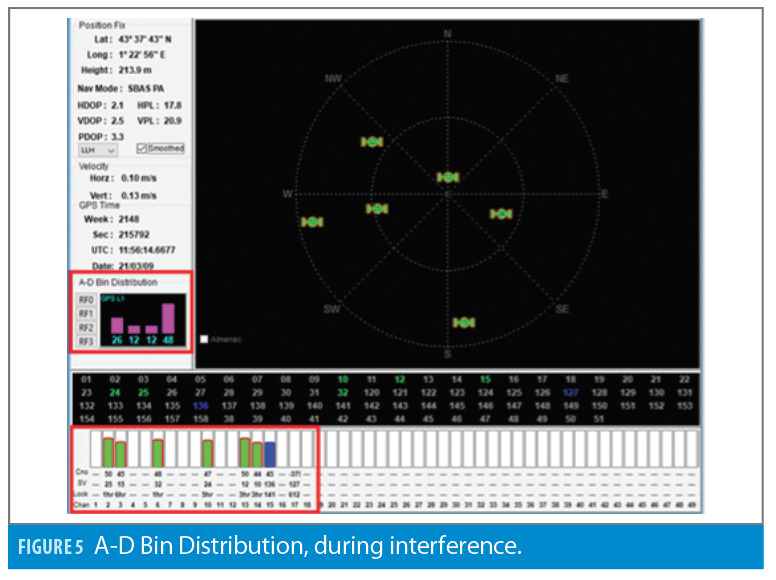
Analysis Method
Step 1: Identify flights with ADS-B Out drops
FlightAware’s Firehose service was used to analyze nine months of historical flight data from September 2020 through June 2021 over the CONUS. FlightAware’s Firehose service was chosen because of its extensive ADS-B coverage, mature Application Programming Interface (API), reliable connection, available technical support, and existing subscription use.
FlightAware fuses aircraft position reports from multiple sources and prioritizes ADS-B Out position reports as the highest priority. ADS-B Out drops can be observed using FlightAware’s web-based flight tracker through the “Reporting Facility” or “Report Update Type.” Figure 6 shows an example flight from the web interface, which experienced an ADS-B Out drop. On this example flight, American Airlines flight 1825 from Phoenix (KPHX) to New York (KJFK) on May 26, 2021, lost ADS-B Out for approximately 10 minutes over the New Mexico and Arizona state border while cruising at 35,000 feet. During the loss of ADS-B Out, FlightAware’s position reports are based on ground radar (Denver Center) and Multilateration (MLAT) sources.
All ADS-B Out drops, such as the example shown in Figure 6, were identified for the entire nine month period over the CONUS. The logic of identifying ADS-B Out drops was automated by querying FlightAware’s Firehose API commands using python scripts to automate the process identified in Figure 7.
Step 2: Identify co-located ADS-B Out drops
An aircraft’s loss of ADS-B Out alone is not an indicator of GNSS interference. There could be malfunctioning transponder equipment, a loss of ADS-B coverage area, intentional power-off of the transponder or GNSS equipment, etc. However, if more than one aircraft experiences an ADS-B Out drop near the same time and location, this rules out aircraft specific equipment as the root cause and points toward external causes such as external RF interference.
For this analysis, ADS-B Out drops identified in Step 1 were compared with one another to identify co-located ADS-B Out drops that occurred within +/- 5 minutes and within 10 nautical mile (NM) horizontal distance to one another. The thresholds of 5 minutes and 10NM were chosen to be overly conservative and pinpoint aircraft that were very close to one another in position and time to avoid false positive interference events.
Figure 8 shows a second example flight with an ADS-B Out drop that meets the 5 minute and 10NM co-location criteria with the example from Figure 6. On this second example flight, Delta Airlines flight 2397 from Los Angeles (KLAX) to Atlanta (KATL) on May 26, 2021, lost ADS-B Out for approximately 30 minutes over the same New Mexico and Arizona state border region while cruising at 37,000 feet. The co-located loss of ADS-B Out function is presumed to be due to external RF interference and was identified as an interference event.
This process of identifying co-located ADS-B Out drops, as illustrated in the examples, was performed for all ADS-B Out drops identified in Step 1.
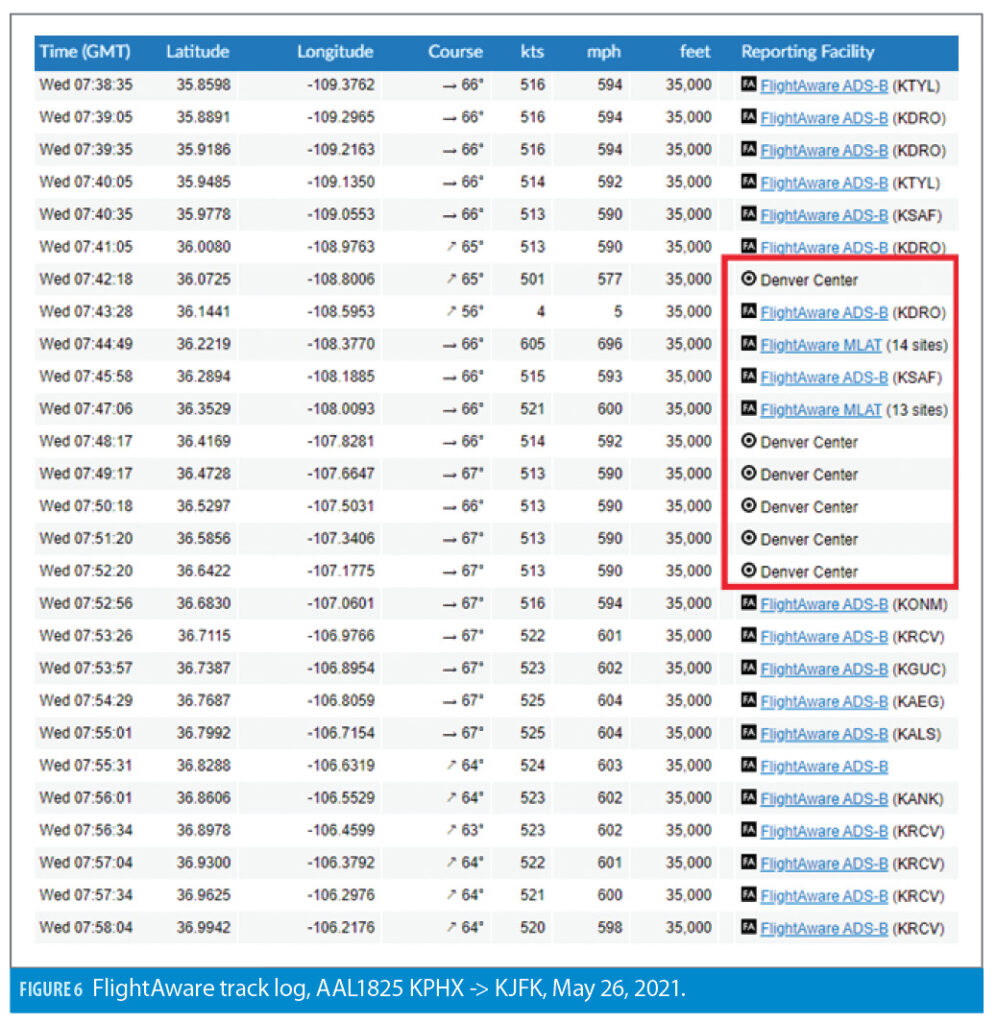
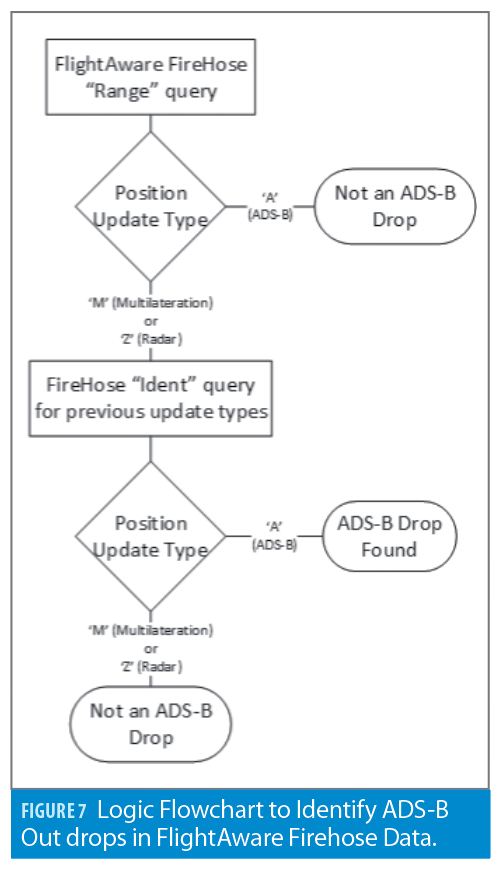
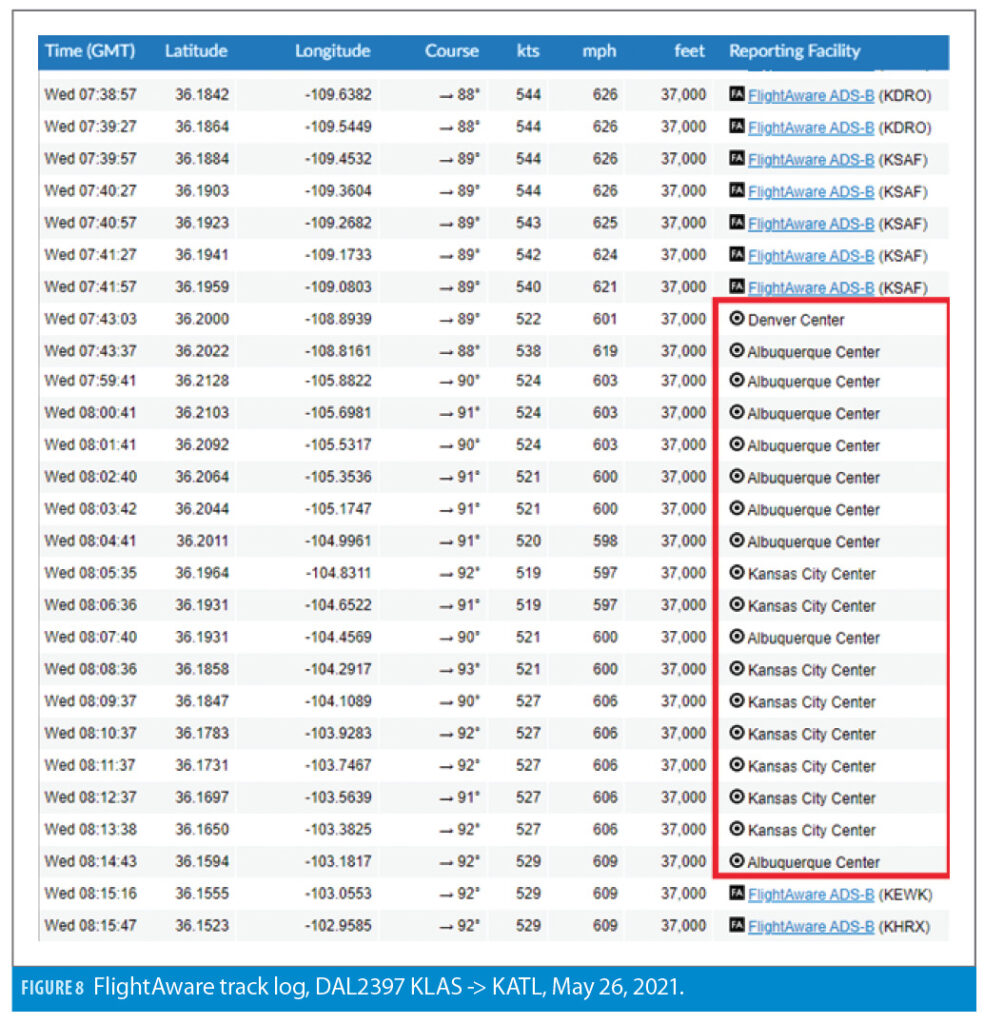
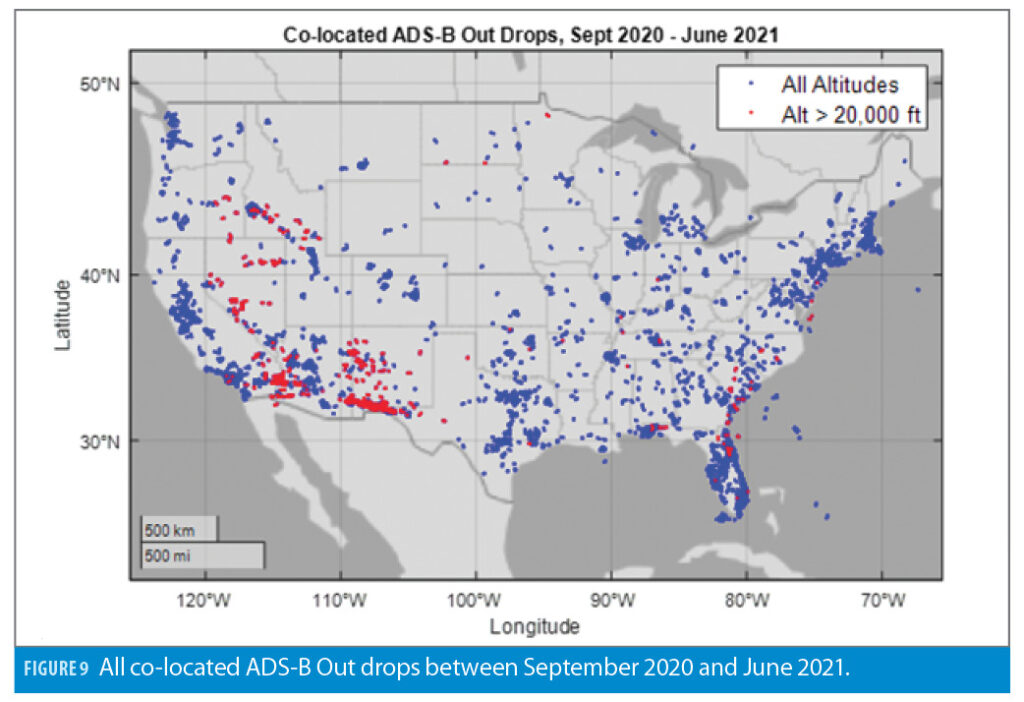
Results
Using the described method, approximately 8,500 interference events were identified over the CONUS during the nine month period from September 2020 through June 2021, each event requiring at least two co-located ADS-B Out drops. The analysis included all commercial, business, cargo and general aviation flights that were equipped with ADS-B Out. Figure 9 shows all nine months’ worth of co-located ADS-B Out drops, or approximately all 8,500 interference events identified using the method described. This figure alone can be misleading for a number of reasons:
1. The higher number of co-located ADS-B Out drops in a region does not necessarily indicate there is worse interference in that area. It could be an indicator of a higher volume of air traffic making it easier to meet the co-located criteria.
2. Conversely, no detected events doesn’t mean there is no RF interference; it could simply indicate there is low air traffic volume or no air traffic at all.
3. Figure 9 does not take into account any time correlation of the events; e.g. one cannot determine if there is repeated daily interference in a geographical location or if the interference was a one-time event.
4. The detection method, and the events plotted in Figure 9, cannot discern between intentional and unintentional interference. It is not uncommon for the United States government to perform intentional GNSS interference testing that can affect airborne GNSS receivers. Many of the detected events are expected due to intentional RF interference published to aircraft operators by GPS Notice to Airmen (NOTAM).
Figure 9 also depicts interference events above 20,000 feet in red. Twenty-thousand feet filters out most general aviation aircraft and is used to validate the assumption that most interference sources are terrestrial based.
To depict the daily occurrences of the results, Figure 10 shows the number of days where interference was detected in a region over the nine month (274 days) time period. To create this heatmap, a 1-by-1 latitude-longitude grid was created. If any co-located ADS-B Out drops were detected over a one day time period in the grid location, it was considered one daily event. Therefore, if there were 100 events, or one event on the same day, they are given the same weight. Figure 10 is a better representation of “how often” events were detected by region than Figure 9.
Figure 11 shows the same daily occurrence information for all interference events detected above altitudes of 20,000 feet, filtering out most general aviation flights, approach and departure flight phases, and potentially highlighting regions with higher power interference.
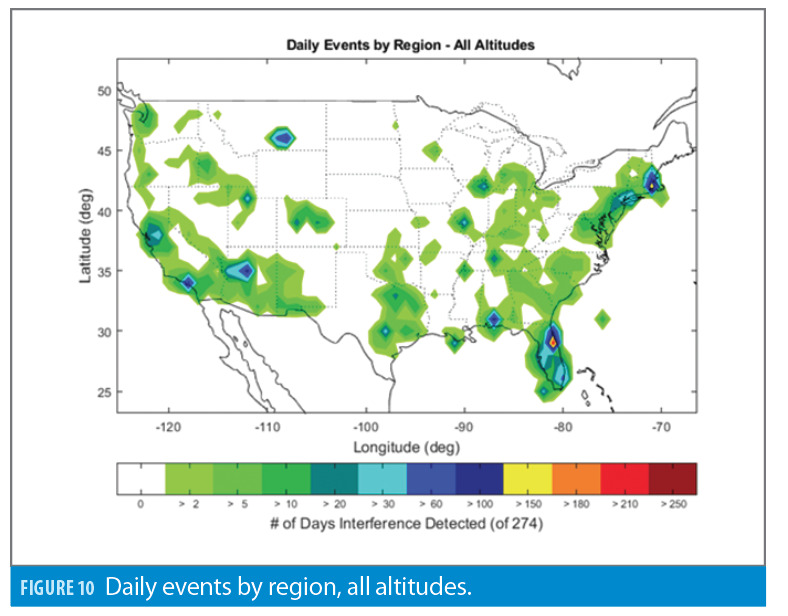
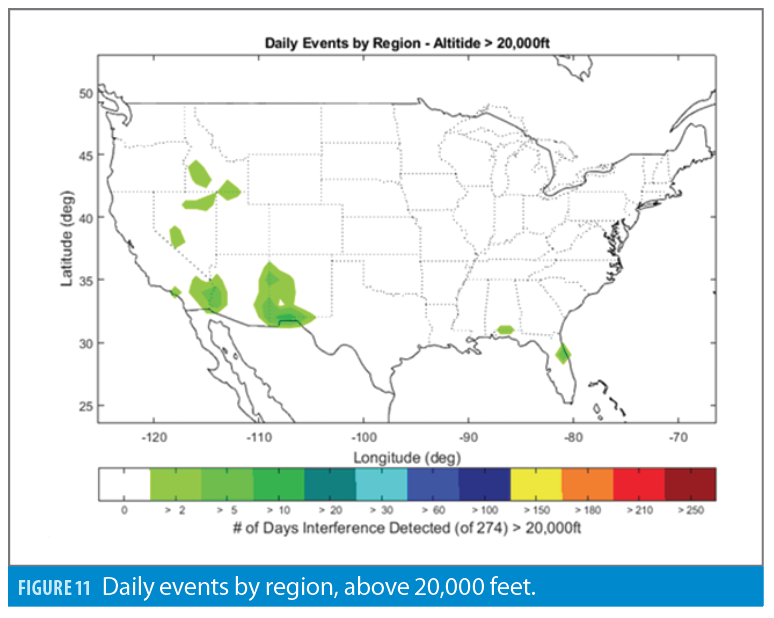
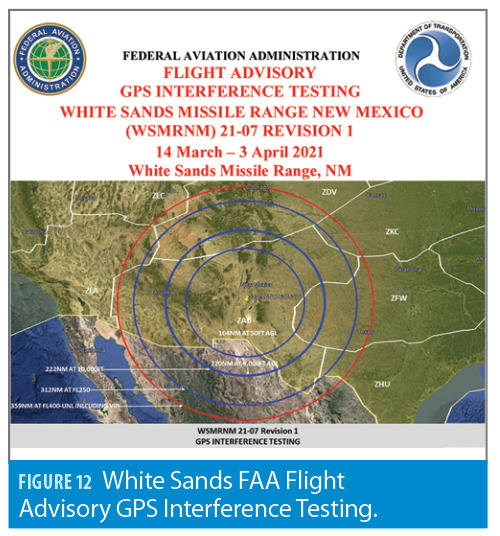
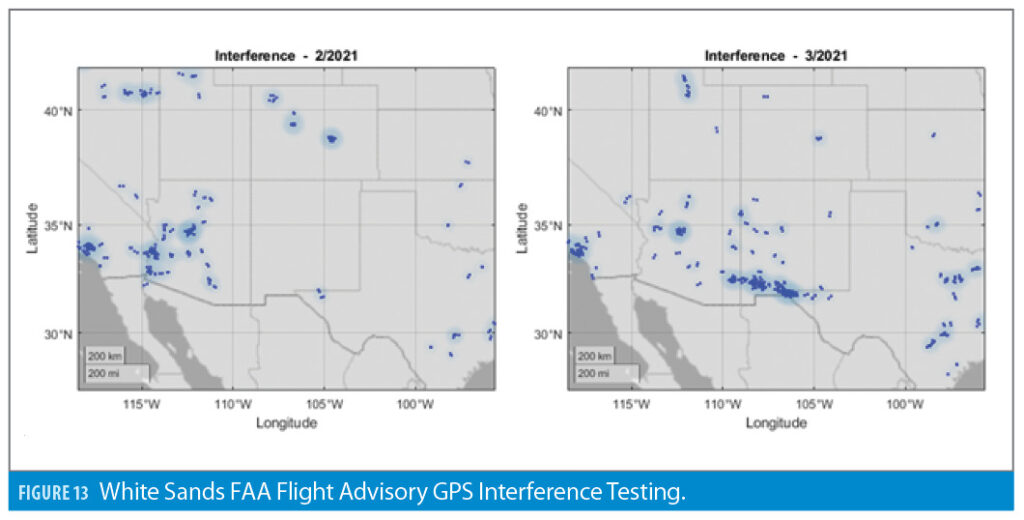
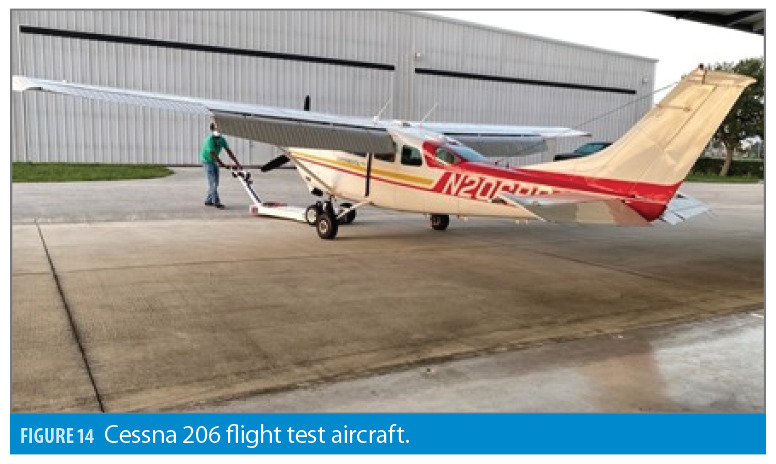
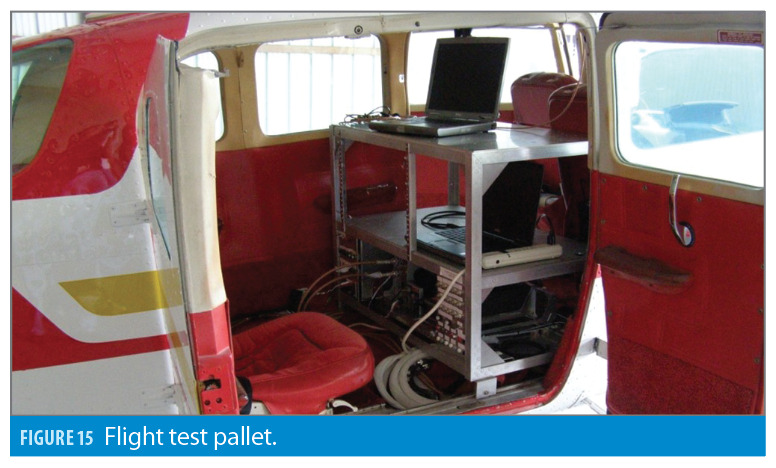
Validation of Results
The results were validated by comparing the detected interference events against times and locations of known intentional RF interference. Figure 12 shows an example FAA Flight Advisory [9] that was issued over the White Sands missile range in New Mexico due to intentional GPS interference testing between March 14 and April 3, 2021.
Figure 13 shows all interference results detected in the same White Sands, New Mexico, region for the entire month of February 2021 on the left and for the entire month of March 2021 on the right. There were virtually no interference events detected in New Mexico in February 2021. A significant amount of interference events were detected in March 2021. Many of the interference results detected in New Mexico during March 2021 were high altitude events, which may be the result of higher power interference.
Figures 12 and 13 show one example of a strong correlation between expected interference and the analysis results. Other similar examples were found, further validating the results.
Follow-up Flight Testing
In February and March of 2022, Collins performed two dedicated flight tests in a Cessna 206 flight test aircraft, pictured in Figures 14 and 15, to further investigate and confirm the airborne GNSS RF interference.
Both flight tests were flown in the vicinity of Daytona Beach, Florida, at or below 4,000 feet MSL where the results of the analysis indicated there is a high probability of encountering airborne GNSS interference. Each flight test was instrumented with the following:
• Collins certified GPS-4000S (GPS + SBAS L1 only)
• Collins prototype DFMC GLU-2100 (capable of tracking GPS L1/L5 + Galileo E1/E5a + SBAS L1/L5 using a Trimble AV39 antenna)
• Non-Collins certified ADS-B Out transponder and GPS (the test aircraft ADS-B out installation)
On both flight tests, airborne GNSS RF interference was confirmed on both Collins receivers by observing the A-D information. These occurrences also coincided with a loss of ADS-B out on the test aircraft (non-Collins) transponder. One of the interference events is detailed here.
Interference Event 1: February 24, 2022
GPS Week: 2198
GPS ToW (s): 401643
Latitude: 29.12401
Longitude: -81.19694
Altitude: 2,000ft MSL
The (2-bit) normalized A-D bin distribution for both Collins receivers is plotted as a time series in Figures 16 and 17 around Interference Event 1. The in-band interference in this example lasts approximately 10 seconds and is similar in duration to all interference events observed on these two dedicated flight tests.
The flight test aircraft also experienced a loss of ADS-B Out reporting at the same time and location. The loss of ADS-B Out from the non-Collins ADS-B out system persisted for approximately 4 minutes in this example. Figure 18 shows the coincident disruption in ADS-B Out reporting from FlightAware. The confirmed interference on these dedicated flight tests provides further confidence that the results of the analysis are valid. The flight tests also provide insight that although the interference observed in the Daytona Beach, Florida, area may be frequent, the events are short in duration.
Because Collins flew both a certified GPS + SBAS L1 only receiver as well as a prototype DFMC (GPS L1/L5 + Galileo E1/E5a + SBAS L1/L5) receiver, it is also worth noting that some of the interference events caused a loss of position from the L1 only receiver, whereas the prototype DFMC receiver retained enough measurements to continue to compute position. Figures 19 and 20 show an example interference event from both receivers and how simply adding dual frequency or multi constellation capability may help prevent loss of positioning during short interference events such as the ones observed on the flight tests.
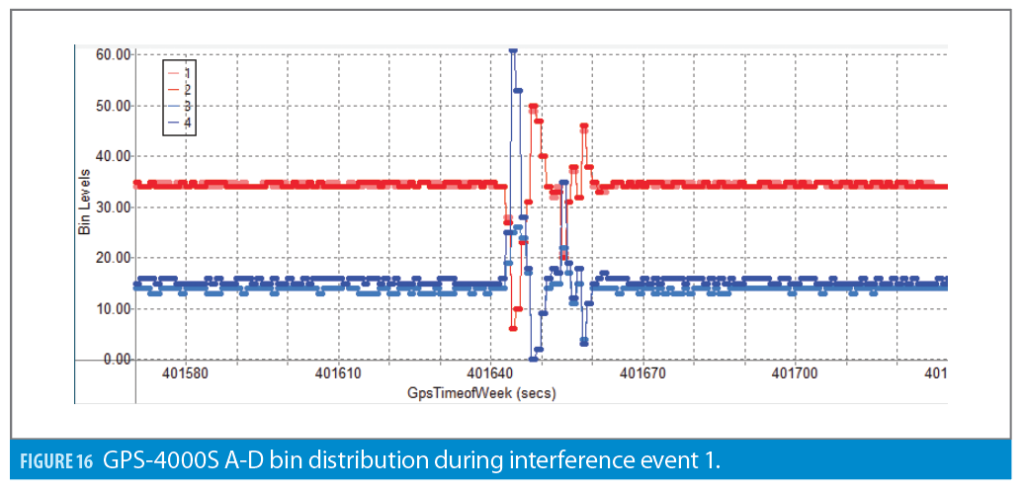
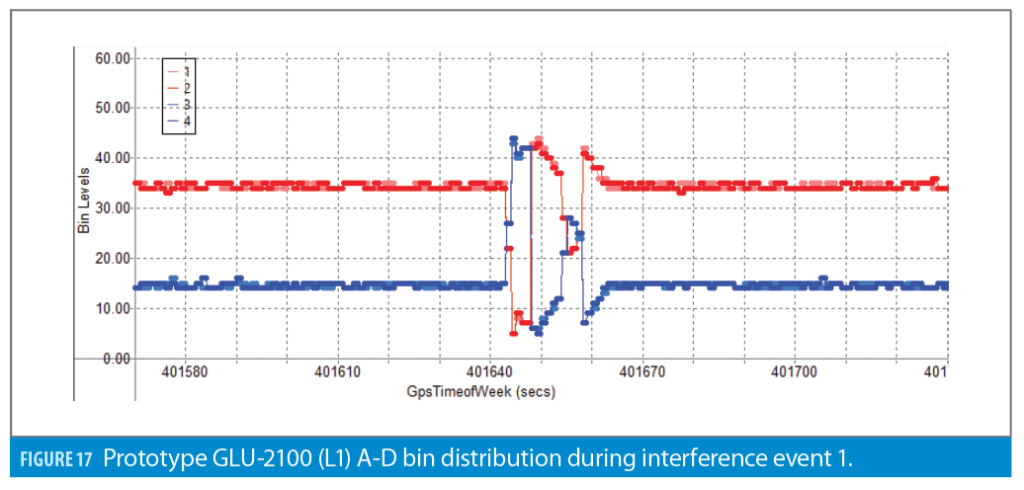
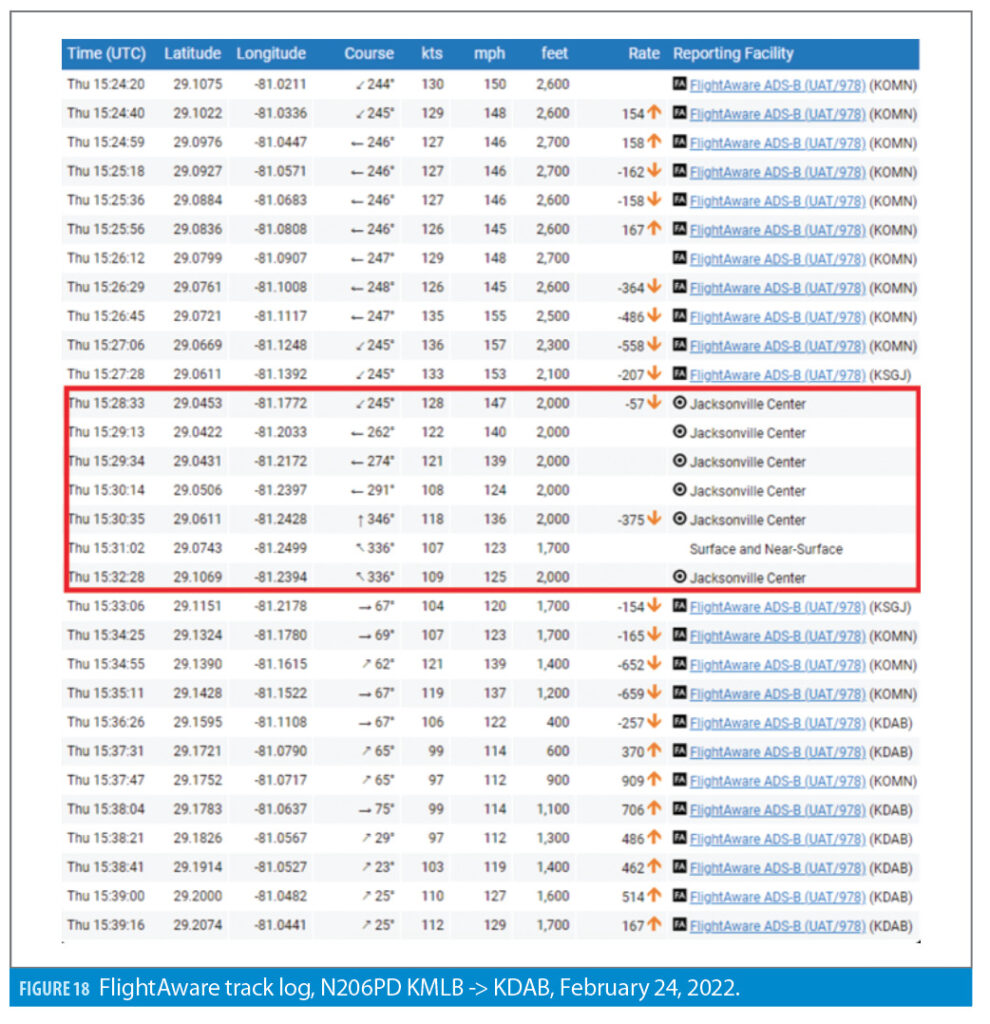
Conclusions
Collins has developed a method to identify airborne GNSS RF interference by detecting ADS-B Out drops from co-located aircraft equipped with ADS-B Out transponders. A toolset to mine FlightAware’s Firehose service was developed and flight data over the CONUS from September 2020 through June 2021 was analyzed and results were mapped. Validation of the results shows the method was successful at detecting RF interference when and where intentional RF interference was expected via aviation NOTAMs. A dedicated flight test campaign was also performed to validate the results and confirm airborne GNSS interference is occurring in the identified regions.
The number of detected interference events is a small fraction of the total number of flights included in the analysis. Less than 1% of all flights over the CONUS during the time period of analysis resulted in a co-located ADS-B drop. The detected interference results represent outliers that the aviation GNSS community should continue to investigate and understand. Future aviation GNSS receiver standards should continue to improve GNSS receiver interference resiliency. The introduction of dual-frequency multi-constellation receivers and the use of the more resilient GNSS signals should help improve the robustness of future aviation GNSS receivers.
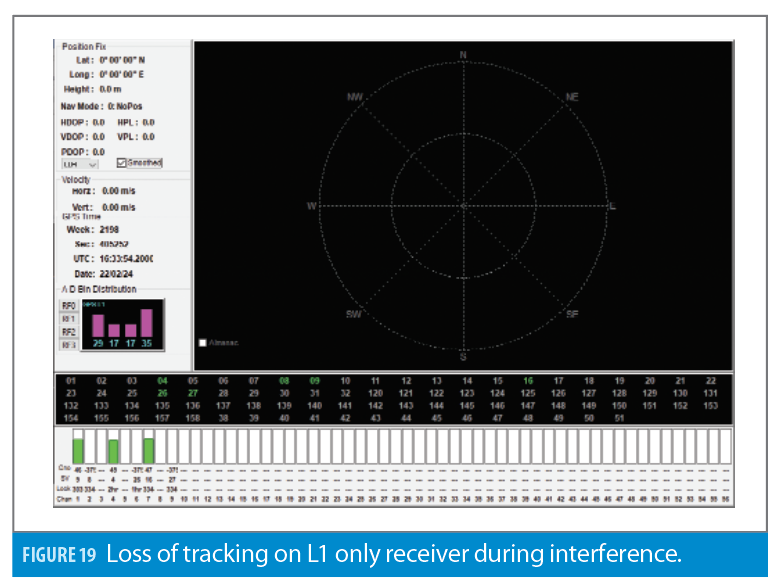
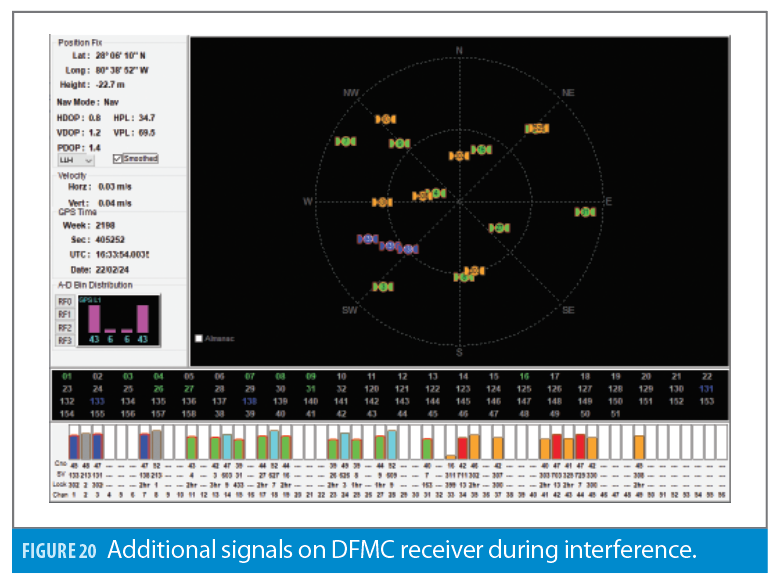
Acknowledgement
This article is based on material presented in a technical paper at ION GNSS+ 2021, available at ion.org/publications/order-publications.cfm.
References
(1) Final Rule, Automatic Dependent Surveillance-Broadcast (ADS-B) Out Performance Requirements to Support Air Traffic Control (ATC), 75 FR 30160, May 28, 2010.
(2) AC 20-165A, Airworthiness Approval of Automatic Dependent Surveillance–Broadcast (ADS-B) Out Systems, November 7, 2012.
(3) https://www.faa.gov/nextgen/equipadsb/installation/equipment/
(4) Darabseh, A., Bitsikas, E., and Tedongmo, B., “Detecting GNSS Jamming Incidents in OpenSky Data,”. EPiC Series in Computing, Volume 67, 2019, Pages 97-108.
(5) TSO C145d, Technical Standard Order: Airborne Navigation Sensors Using The Global Positioning System Augmented By The Satellite Based Augmentation System (SBAS), December 20, 2013.
(6) TSO C145e, Technical Standard Order: Airborne Navigation Sensors Using The Global Positioning System Augmented By The Satellite Based Augmentation System (SBAS), May 5, 2017.
(7) TSO C146e, Technical Standard Order: Stand-Alone Airborne Navigation Equipment Using The Global Positioning System Augmented By The Satellite Based Augmentation System (SBAS), May 5, 2017.
(8) TSO C161a, Technical Standard Order: Ground Based Augmentation System Positioning and Navigation Equipment, December 17, 2009.
(9) Flight Advisory GPS Interference Testing White Sands Missile Range New Mexico (WSMRNM) 21-07 REVISION 1. March 10, 2021.
Authors
Jeremy Kazmierczak
is a Sr. Principal Systems Engineer for Collins Aerospace, Commercial Systems Navigation Avionics. Jeremy is currently working on developing Collins’ next generation of commercial aviation GNSS products and has previously supported the development and certification of other aviation navigation avionics such as Radio Altimeter and Distance Measuring Equipment. Jeremy holds a Bachelor’s degree in Aerospace Engineering from Embry-Riddle Aeronautical University and is an FAA private and instrument rated pilot.
Angelo Joseph is a Director, Systems Engineering for Collins Aerospace, Commercial Systems Navigation Avionics. At Collins, Angelo currently leads the team that develops the next generation GNSS products and is a Principal Architect involved in product development, innovation and strategy. Angelo is involved in all aspects of GNSS receiver design and development including systems, hardware, RF and software. His innovative GNSS receiver design won him the prestigious Collins “Engineer of the Year” award in 2017. Prior to joining Collins, Angelo worked as GPS Engineer at Avidyne Corporation. At Avidyne, Angelo was responsible for designing and developing Avidyne’s first SBAS Beta-3 airborne GPS receiver. Prior to his stint at Avidyne, Angelo was a Senior GPS Systems Engineer at NovAtel in Calgary, Canada. He was a core member of the team that developed the WAAS-G2 ground reference receiver. In addition, he played key roles in the initial design of NovAtel’s GPS/Galileo ground reference receivers and the first generation EGNOS, MSAS and WAAS ground reference receivers. He was a co-inventor of the SafeTrack technology at NovAtel. He has multiple GNSS patents and a Masters in Geomatics Engineering from the University of Calgary.
George Cook is a Principal Systems Engineer for Collins Aerospace, Commercial Systems Navigation Avionics. George is currently working on developing Collins’ next generation of commercial aviation GNSS products. Prior to working at Collins, George worked with GNSS based asset tracking in the shipping and recreational golf industries. George is a licensed Professional Engineer (PE) in Florida and holds a Bachelor of Science degree in Electrical Engineering from the University of Central Florida.