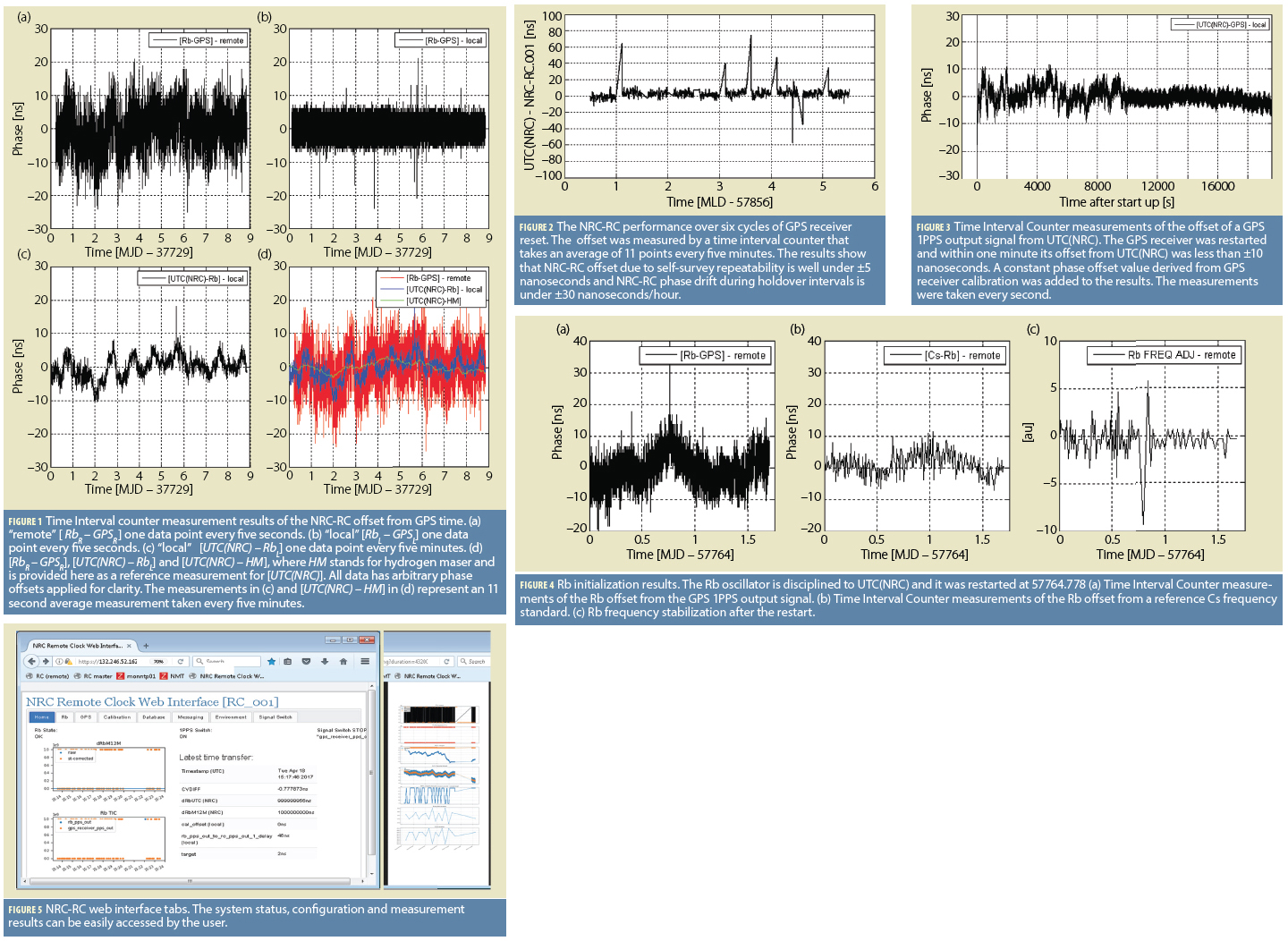
Recent changes in financial market regulations, as well a growing demand from traders and service providers for more accurate time, have driven the development of commercial time services with sub-microsecond precision. Several National Metrology Institutes (NMI), including NRC, are currently offering or are developing such services (as described by M. Lombardi et alia and P. Tavella et alia in Additional Resources near the end of this article). The NMIs’ advantages in providing time services are their expertise in time transfer and metrology, and the authority they have in their respective countries for the realization and dissemination of official time.
Most NMI’s contribute to the production of Coordinated Universal Time (UTC) through regular reporting of their timescales to the Bureau International des Poids et Mesures (BIPM). While UTC is a post-processed, paper timescale, each contributing laboratory produces its own version of UTC, represented by UTC(k), which it disseminates in real time. Regular reports from the BIPM to the contributing laboratories of the offsets between UTC and UTC(k) provide traceability of the UTC(k) timescales to UTC. Over decades, NMIs have perfected the methods and tools for the highest accuracy time synchronization. Now this knowledge is being adapted to services for government, industry and military applications.
NRC has developed a Remote Clock product (NRC-RC) that disseminates time traceable to UTC(NRC) with sub-microsecond precision. The NRC-RC provides superior-accuracy time to its client’s system that guarantees traceability down to the end users’ devices. The service is robust, reliable, and secure. Based on a high quality local oscillator and GPS for time transfer, the remote clock is continuously monitored and adjusted to UTC(NRC) to ensure traceability and minimize vulnerability to GPS signal jamming and spoofing.
Results and Discussion
The NRC-RC configuration consists of a high quality rubidium (Rb) frequency standard, a GPS receiver, a time interval counter, a control computer, and several auxiliary components. Such systems are installed at the remote client site, and at the NRC main laboratory. The NRC-RC output signals consist of a one pulse per second (1PPS) signal, representing UTC(NRC-RC), and a timestamp provided in one of the standard NTP or PTP servers ASCII formats. An additional sine signal output at 5 or 10 megahertz, syntonized to UTC(NRC-RC), is also available.
Several measurements are performed at each NRC-RC with periods ranging from seconds to minutes. The time offset of the NRC-RC Rb standard 1PPS is measured against the 1PPS from GPS time as derived from the receiver, [Rb – GPS]. The GPS receiver data of the time offset between the receiver time (its 1PPS) and the time from each satellite, GPSsd is also recorded. Similar setup is installed at the NRC main laboratory where the Rb 1PPS signal offset from UTC(NRC), [UTC(NCR) – Rb], is also measured.
Every few minutes, the data acquired at the NRC main laboratory (local, subscript L) is transferred to the RC site (remote, subscript R) where time and frequency transfer calculations and UTC(NRC-RC) timescale adjustments are made. The time transfer between NRC-RC and UTC(NRC), [RCR – UTC(NRC)], is shown in Equation 1. It consists of all these measurement results and also contains the common view comparison of the GPS data. It includes a term equal to the average offset of GPS time from receiver time for each satellite that is visible simultaneously at NRC and at the remote site, [GPSsdR – GPSsdL]. The common view method effectively removes the GPS timescale from the equation. By comparing the offset information collected, the Rb frequency and 1PPS are adjusted to keep UTC(NRC-RC) time at the remote site within a few nanoseconds of UTC(NRC). Calibration offset value, D, is added to compensate for static cable and instrumentation delays.
[RCR – UTC(NRC)] = [RbR – GPSR] + [GPSsdR – GPSsdL] – [RbL – GPSL] – [UTC(NRC) – RbL] +D [1]
The measurement results for [RbR − GPSR], [RbL – GPSL] and [UTC(NRC) – RbL] are shown in Figure 1 (a), (b) and (c) (see inset photo, above right, for all figures), respectively. The “local” implementation of NRC-RC does not apply the time transfer information ([GPSsdR – GPSsdL] − [RbL – GPSL] − [UTC(NRC) – RbL]) to the Rb disciplining, effectively keeping it locked to the GPS signal with a time constant of tens of seconds. As a result, the [RbL – GPSL] measurement shows a straight line behavior, with short-term noise, as can be seen in Fig. 1(b). The fluctuations in [UTC(NRC) – RbL] shown in Fig. 1(c) are due to the GPS time signal variation with respect to UTC(NRC). Except for differences in short-term noise, Fig. 1(d) clearly shows that [Rb − GPSR] is equivalent to [UTC(NRC) − RbR] in the intermediate and the long term. Since RbL is equivalent to GPSL (Fig 1(b)), and as it clearly follows from Equation 1, RbL is locked to UTC(NRC). Therefore, UTC(NRC-RC) that is derived from RbR 1PPS follows UTC(NRC). In Fig. 1(d) we also show for comparison hydrogen maser, HM, offset from UTC(NRC) to demonstrate the noise characteristics of the UTC(NRC) signal and the measurement system.
In order to achieve time transfer accuracy within a few nanoseconds with respect to UTC(NRC), we calibrate internal delays for each individual component of the NRC-RC system, such as the 1PPS distribution system, the Time Interval Counter, and the GPS receiver 1PPS output. For GPS receivers it is important to use one standard configuration for all receivers at different NRC-RC sites to ensure optimal common view noise cancellation. Ideally the NRC-RC system should be calibrated with the GPS antenna that is used on site, but this is not always feasible due to specific site circumstances and accessibility issues. In these cases, in order to achieve the best uncertainty, the GPS output signal should be calibrated using time transfer with a traveling caesium frequency standard (Cs). Using regular performance Cs we achieved time transfer uncertainty with a remote NRC-RC site of under ±10 nanoseconds.
One important parameter for a remote time dissemination system is its hold over time. We use a high quality rubidium oscillator and we adjust its magnetic field setting to control the frequency and keep it close to UTC(NRC). When, for any technical reason, the GPS signal or the time transfer information from the NRC main lab is unavailable or deemed unreliable by the NRC-RC algorithm, the Rb oscillator is allowed to free run for a period of time determined by its stability and the level of drift in UTC(NRC-RC) that is acceptable for the application.
Another contributor to the overall uncertainty of the NRC-RC time signal is the accuracy and the reproducibility of the GPS receiver self-survey position. We tested both the NRC-RC holdover and the signal stability over several cycles of NRC-RC GPS receiver resetting. We used the NRC-RC.001 unit, connected to a small antenna, collocated with UTC(NRC). This way the NRC-RC.001 signal accuracy can be evaluated with lowest uncertainty. Figure 2 shows the results for [UTC(NRC) − NRC-RC.001] offset measured with the time interval counter. The hold over periods for each self-survey cycle lasted for 10,000 seconds. The offset level change due to the variation in the GPS receiver-determined antenna position is well under ±5 nanoseconds. During the holdover intervals the Rb oscillator inside NRC-RC.001 was free running and as it can be seen from the figure, the offset of NRC-RC.001 never exceeded ±80 nanoseconds.
The corresponding Rb phase drift rate of ±30 nanoseconds/hour will be improved in the next upgrade to NRC-RC where additional fine scale adjustments of the Rb magnetic field will be implemented.
The NRC-RC system can be used in a stationary setup or as a traveling calibration system. If the system needs to be restarted, it is important that the initialization time required to bring the system operating parameters within the specification range is short. If the site location has not been surveyed before and the GPS antenna coordinates are not known, several hours may be required by the GPS receiver used in the NRC-RC system to complete the self-survey and obtain accurate antenna coordinates. We analyzed the receiver performance after the cold start and measured its 1PPS output signal offset from UTC(NRC). The results are shown in Figure 3. The GPS time 1PPS signal offset from UTC NRC is less than ±10 nanoseconds of its fully surveyed value within one minute after start up. The site survey by the GPS receiver is completed after approximately 10,000 seconds. It results in an improved signal-to-noise ratio as can be seen in the plot, but does not affect the time offset measurements significantly.
Another important contributor to the start-up process is the Rb standard. Its frequency and 1PPS signal output time have to be adjusted based on the time transfer information. It is important to bring the frequency of the Rb close to UTC(NRC) as fast as possible, because it directly impacts the holdover time of the unit. In Figure 4 we show the Rb 1PPS signal offset from GPS receiver time and from the reference Cs standard following Rb start up. The Rb was restarted at MJD = 57764.778 and the corresponding spike can be seen in the [RbR − GPSR] data shown in Fig. 4(a). The [CS − RbR] data, shown in Fig. 4(b) that is being collected every 10 minutes doesn’t show any phase jumps indicating immediate recovery of accurate time by the Rb after the start up. The curve shown in Fig. 4(c) represents the Rb frequency adjustment values. It can be seen that the frequency adjustment algorithm takes only three steps to bring the Rb frequency to a stable value, in the current implementation of the Rb control algorithm. In the future we plan to modify the Rb control algorithm to shorten the frequency adjustment period after Rb start up to just a few minutes.
For reliable and secure operation of the NRC-RC system we introduced watchdog monitoring of critical system parameters such as system temperature, power levels, equipment health and others. Alerts and notifications are generated by the watchdog and, in some cases when the algorithm detects a major problem with data and deems the output signal quality and accuracy unreliable, the output time signals from NRC-RC are stopped to prevent the dissemination of inaccurate time.
NRC-RC reduces the vulnerability of time dissemination to GPS signal jamming and spoofing. One of the important watchdog process functions is to detect GPS signal jamming or other malfunction and stop the GPS data from being used for time transfer until the interference stops. Due to the long holdover times allowed by the NRC-RC performance, as was shown in Figure 2, the NRC-RC has high tolerance to GPS signal jamming.
In many cases the NRC-RC system has to be installed at an unmanned facility. For this reason it is important to have the full control of the remote system integral parts for power cycling, configuration parameters adjustment, data logging and environmental monitoring. We implemented a web-based interface for easy control and monitoring. Two tabs from the web interface are shown in Figure 5.
Conclusion
We have developed an affordable reliable secure system that disseminates traceable time with uncertainties in tens of nanosecond range.
Careful calibration of the NRC-RC system and time transfer uncertainties allow for long holdover times. With the rubidium oscillator that we selected for this product, 95% guaranteed uncertainty is less than ±200 nanoseconds for eight hours of autonomous operation. Smaller uncertainty or longer holdover times can be achieved with higher quality and more expensive atomic standards. The control systems integrated in the RC algorithm alert the client and NRC staff if any system disruptions or anomalies occur. Events, such as GPS signal degradation, loss of Ethernet connectivity, changes in the SNR level that can be caused by jamming or spoofing, etc., are all communicated via alerts such as email messages, flashing lights or other notifications.
The NRC-RC system is able to take pre-programmed corrective measures based on severity of the problem. The auxiliary systems of the NRC-RC provide: a timecode signal that, along with 1PPS, can be used as a stratum-0 clock for the local NTP or PTP network; a monitoring and alert system for traceable time dissemination across a local network; system operation watchdog processes; and other technical and support services to ensure system security and reliability. Future upgrades include upgrades to system hardware and software to allow time transfer via GLONASS and Galileo GNSS systems.
Several NRC-RC systems can be installed at the client site to improve redundancy and reliability and reduce uncertainty within the system. Each system provides timecode, 1PPS, and frequency (e.g. 10 megahertz) signals traceable to UTC(NRC), UTC and SI Proper Time Frequency. The use of the NRC-RC with each grandmaster of the client’s PTP network, along with the continuous monitoring and analysis of the PTP performance of their clients’ clocks, ensures the quality of the local network systems and provides the necessary evidence to demonstrate time synchronization to UTC for regulatory purposes, while guaranteeing sub-microsecond accuracy across the client’s network and globally between different networks around the world.
Acknowledgement
This article is based in large part on a paper presented at the ION PTTI 2017 conference, January 30-February 2, 2017 in Monterey, California.
Additional Resources
[1] Lombardi, M., A. Novick, G. Neville-Neil, and B. Cooke. “Accurate, traceable, and verifiable time synchronization for world financial markets.” Journal of research of the National Institute of Standards and Technology 121 (2016): 436-463.
[2] Tavella, P., I. Sesia, G. Cerretto, G. Signorile, D. Calonico, E. Cantoni, C. De Stefano, V. Formichella, R. Costa, P. Cerabolini, L. Rotiroti, A. Simonetti, A. Colombo, P. Defraigne, N Ozdemir, M. Gandara, P. L. Puech, V. Hamoniaux, E. Varriale, Q. Morante, T. Widomski, J. Kaczmarek, J. Uzycki, K. Borgulski, P. Olbrysz, J. Kowalski, A. Cernigliaro, F. Fiasca, A. Perucca, A. Samperi, V. Dhiri, E. Giulianini, M. T. Veiga, T. Suárez, M. Mangiantini, A. E. Wallin, L. Galleani, D. Hindley. “The Horizon 2020 DEMETRA project: DEMonstrator of EGNSS services based on Time Reference Architecture.” Metrology for Aerospace (MetroAeroSpace), 2015 IEEE. IEEE, 2015.