In a race with the U.S. to develop a laser communications network in space, China’s BeiDou GNSS has conducted an inter-satellite and satellite-ground station experiment using using lasers rather than the usual radio signals. The technology could potentially transmit data a million times faster than by radio signal to almost any location. Some experts say it could increase satnav accuracy by a factor of 6 to 40 by synchronizing the satellites’ atomic clocks with laser beams.
[Image courtesy China Daily.]
Researchers at the Changchun Institute of Optics, Fine Mechanics and Physics in the northeastern province of Jilin developed a shape-changing mirror telescope that it at the heart of the method. It could enable a satellite to beam data to the ground or to another satellite at several gigabytes per second, rather than kilobytes by radio frequency. BeiDou’s fastest communication performance in the experiment is classified.
In a competing but lagging development, the U.S. National Aeronautics and Space Administration (NASA) announced on November 29 that a December 4 launch of an experimental satellite will lead to similar experiments, testing data transmission via laser beam at 2.8GB per second. This comes after a two-year delay in the project.
BeiDou and all other GNSS satellites normally inter-communicate by radio signal, which can transmit only short text messages because of limited bandwidth. With lasers, a space network could transmit data a million times faster. Laser communication offers wider bandwidth, is less vulnerable to cyber-espionage, and uses lighter, more compact technology.
A ground station for laser communication is usually a fixed facility with sophisticated devices including a large telescope, a beam tracking and locking unit, and equipment for signal processing. However, the Changchun Institute scientists and engineers packed reception equipment into a car for mobile deployment.
Laser-beam bandwidth can reach one terabyte (1,000GB) per second. Lasers are also less susceptible to being tapped by a third party, and more difficult to jam during electronic warfare than microwaves. A traditional communication satellite is usually bulky, because it requires huge antennas and lots of power to generate and transmit large numbers of radio signals. Laser devices are smaller and lighter, potentially allowing satellites built mainly for other purposes to establish high-speed communication with one another or to the ground, according to Chinese news agency reportage.
Since the 1960s, programs in the U.S., Japan and Europe have sought to develop a laser communication satellite but failed to solve some of the practical problems involved. One of these is presented by the atmosphere: air molecules can absorb or reflect light, so that too few light particles reach their destination. Turbulence can also distort or shake the laser beams so that the light signals became too blurry to read, especially in urban areas with intense human activity. The Chinese researchers in Shenyang have reportedly developed a telescope mirror that can change shape with an electric charge, to reduce the blurring effect caused by air turbulence.
Mozi, the world’s first quantum satellite, reached a download speed of 5.1GB per second in 2016 using laser equipment. Subsequently, Chinese space laboratory Tiangong 2 conducted the world’s first space-ground laser communication in daytime, overcoming another major obstacle in practical application. In 2019, a Chinese laser ground station downloaded data from a satellite at 10GB per second.
NASA’s Laser Communications Relay Demonstration
As space missions generate and collect more data, the need for enhanced communications capabilities has become pressing. Optical communications will increase bandwidth by 10 to 100 times over radio frequency systems, according to NASA statements. Additionally, optical communications provides decreased size, weight, and power requirements, for less drain on spacecraft batteries.
The Laser Communications Relay Demonstration (LCRD) payload includes two optical modules, which generate the infrared lasers that transmit data to and from Earth. The LCRD payload will be ride onboard the U.S. Department of Defense’s Space Test Program Satellite 6 (STPSat-6), scheduled for launch on December 4. LCRD will send test data to and from its ground stations.
Eventually, other missions in space will send their data to LCRD, which will then relay the data down to designated ground stations on Earth. LCRD creates a continuous path for data flowing from missions in space to ground stations on Earth, making a complete end-to-end system. Additionally, LCRD’s ability to both send and receive data from missions and the ground stations makes the system two-way.
Optical signals cannot penetrate cloud coverage, so NASA must build a system flexible enough to avoid interruptions due to weather. LCRD will transmit data received from missions to two ground stations, located in Table Mountain, California, and Haleakalā, Hawaii. These locations were chosen for their minimal cloud coverage. LCRD will test different cloud coverage scenarios, gathering valuable information about the flexibility of optical communications.
Future missions that decide to use optical communications could potentially use LCRD as their relay. One of LCRD’s first operational users will be the Integrated LCRD Low-Earth Orbit User Modem and Amplifier Terminal (ILLUMA-T), a payload that will soon reach the International Space Station. The terminal will receive high-resolution science data from experiments and instruments onboard the ISS and transfer the data to LCRD, which will then transmit it to a ground station, relaying on to mission operation centers and scientists.
In January 2020, the LCRD flight payload was delivered to Northrop Grumman’s facility in Sterling, Virginia, for integration onto STPSat-6. Throughout the development process, the NASA LCRD team worked closely with the Space Force as well as commercial partners like Northrop Grumman and the Massachusetts Institute of Technology (MIT) Lincoln Laboratory.
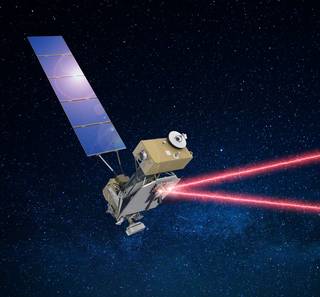
Credit: NASA’s Goddard Space Flight Center
With LCRD relaying data for ILLUMA-T, this will be the first operational optical communications system for human spaceflight. ILLUMA-T will send data to LCRD at rates of 1.2 gigabits per second over optical links, allowing for more high-resolution experiment data to be transmitted back to Earth.