The Joint Research Centre of the European Commission tested seven advanced alternative sources of positioning navigation and timing (A-PNT) platforms through a performance assessment campaign, proving there are viable alternatives to GNSS available in the commercial market today.
LUKASZ BONENBERG, BEATRICE MOTELLA, MATTEO PAONNI, JOAQUIM FORTUNY-GUASCH, EUROPEAN COMMISSION, JOINT RESEARCH CENTRE (JRC), ISPRA, ITALY
IGNACIO ALCANTARILLA MEDINA, EUROPEAN COMMISSION, DIRECTORATE GENERAL DEFENCE AND SPACE, BRUSSELS, BELGIUM
Given the huge benefits brought by Global Navigation Satellite Systems (GNSS) and their widespread use, any disruption would have an outsized effect on widely used services, including those that are life and safety critical. Because of this, the availability of backups or alternative sources of positioning navigation and timing (A-PNT) should be considered a priority.
In the framework of a call for tender launched by the European Commission in December 2020, a performance assessment campaign on a total of seven state-of-the-art A-PNT demonstration platforms was conducted at the Joint Research Centre of the European Commission. The results prove that mature commercial A-PNT technologies that can deliver positioning and/or timing information independently of GNSS already exist in the commercial market.
GNSS Resilience and A-PNT
Today, many economic sectors are strongly reliant on GNSS. This is a measure of the success of global navigation systems but also an indication of a potential vulnerability. Examples of sectors showing a strong reliance on GNSS include transport (air, sea and road), industry (telecom, energy and financial), agriculture, fisheries, and security and defence. The widespread use of GNSS as the sole (or at least primary) source of PNT services in critical infrastructures poses a risk, despite the evident and indisputable benefits brought by the global availability of such services. This makes the availability of A-PNT a must. A-PNT platforms would increase the robustness of GNSS and, interestingly, facilitate their augmentation, leading to an overall performance improvement.
The resilience of PNT technologies should be considered a stringent requirement in many application domains. To enhance the resilience of PNT and GNSS infrastructures and services in the European Union (EU), the European Commission (EC) has taken various actions. Among them:
• Adopting the EU Space Programme (Regulation 2021/696) aiming to preserve EU leadership in space, the Directive (EU) 2022/2557 on the resilience of critical entities and the Directive (EU) 2022/2555 on measures for a high common level of cybersecurity across the Union.
• The publication of the European Radio Navigation Plan (ERNP)[1], which presents the evolution of the landscape of PNT infrastructures in the EU, including new emerging systems that deliver PNT and can complement GNSS services.
• Making new Galileo services, such as the Open Service Navigation Message Authentication (OSNMA) [1], the data authentication function for the Galileo Open Service, freely accessible to users.
With the goal of strengthening the resilience of positioning technologies [2], it is fundamental to ensure interoperability not only among different GNSS, but also between GNSS and other PNT systems. Examples of A-PNT candidates are numerous nowadays and some of them may be integrated within GNSS user terminals. Not all A-PNT technologies were tested in the A-PNT test campaign. A non-exhaustive list of those not tested includes:
• The use of signals from terrestrial communication systems, such as Wi-Fi, Bluetooth and cellular networks, is of special interest to provide additional ranging measurements for trilateration. However, the lack of information from these terrestrial transmitters, such as the transmitter position or a common timing source, may prevent high-accuracy and reliable positioning [6, 7]. 5G NR (New Radio) is the fifth generation of mobile networks, coming after previous generations with an improved level of performance and efficiency. Even though 5G may use some of the same positioning methods as 4G, new frequency bands make it significantly more accurate with lower latency in communications [8].
• The enhanced Loran (eLoran) is the latest in the longstanding series of Loran systems. eLoran evolved from Loran-C, in response to the 2001 Volpe Report[2]. It improves upon previous Loran systems with updated equipment, signals and operating procedures. Such improvements provide better performance and additional services when compared to Loran-C, and enable eLoran to serve as a backup to satellite navigation in many important applications, i.e., Maritime Harbour Entrance and Approach (HEA), Aviation Non-Precision Approach (RNP 0.3), and Timing [9,10].
• Ranging Mode (R-Mode) using signals independent of GNSS is considered one potential source of resilient PNT services. R-Mode is a maritime terrestrial navigation system to employ existing maritime service signals as signals-of-opportunity (SoO) for ranging. It enables positioning and timing for distances of up to a few hundred kilometers from the shoreline, conditioned on the availability of transmitting stations, and bridges the periods when GNSS is unavailable [11].
Given the variety of A-PNT technologies presented, the Directorate General for Defence Industry and Space (DEFIS) of the European Commission (EC) launched the call for tender (CfT) DEFIS/2020/OP/0007 in December 2020, with the objective of assessing the performance of the mature A-PNT technologies capable of delivering accurate and robust positioning, and/or timing services, independently from GNSS. Work was mostly carried out with the scientific and technical lead of the EC Joint Research Centre (JRC).
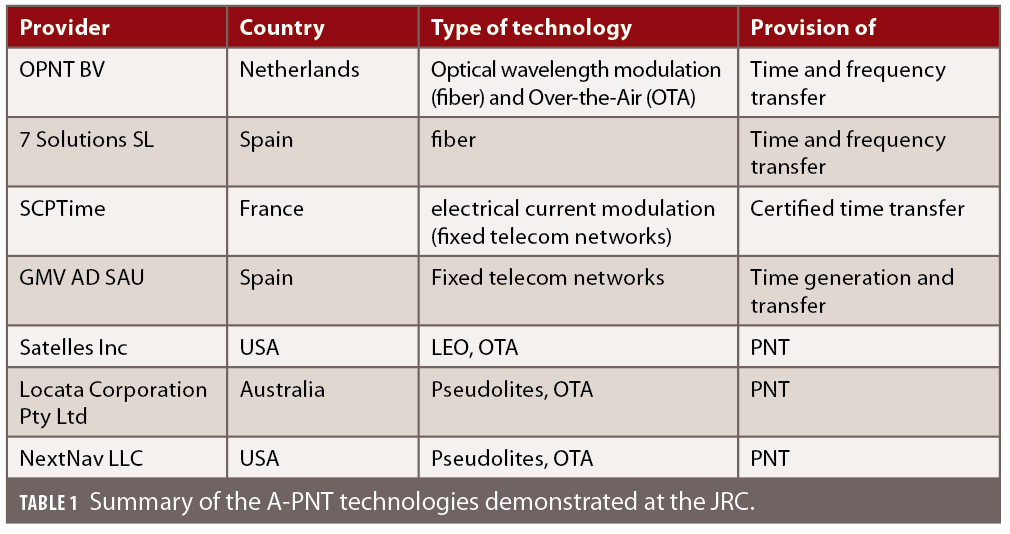
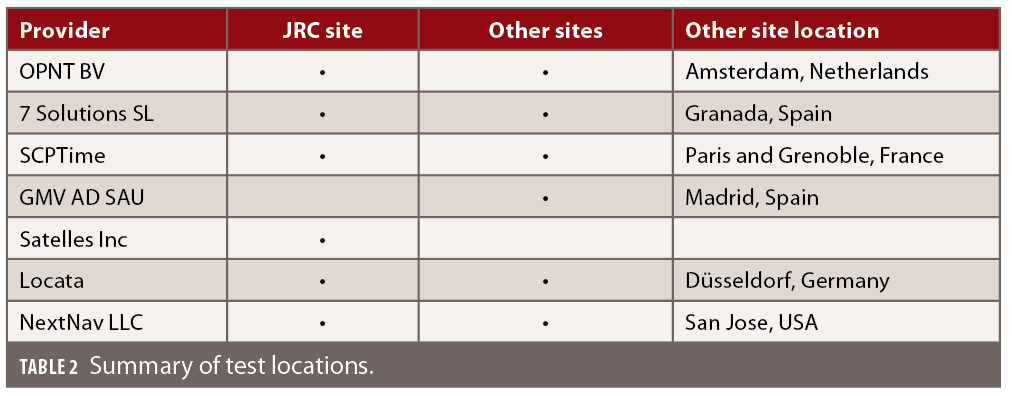
The Tender: Goal and Project Deployment
The main goal of the tender was to analyze technologies that could deliver positioning and/or timing information independently from GNSS and serve as effective backups in the event of GNSS disruption, even in unlikely situations, and, if possible, to provide PNT in environments where GNSS service cannot be efficiently delivered, including urban canyons, indoor, underground, underwater, and fast-moving platforms such as spacecrafts and launchers.
Selected Providers
Four selected providers demonstrated timing services (OPNT, 7 Solutions SL, SCPTime and GMV), and three other providers exhibited both positioning and timing services (Satelles, Locata and NextNav). A summary of the A-PNT demonstration platforms and technologies sustaining them is given in Table 1.
Tested technologies provide a mix of terrestrial resilient time and frequency distribution (optical, electrical and Over The Air, OTA) up to a 105 km OTA time transfer and low Earth orbit (LEO) based services. Assured position was tested using a network of terrestrial transmitters and LEO.
Key Performance Indicators
The tender [12] defined certain specific Key Performance Indicators (KPIs) to comply with. The PNT technologies had to:
• Deliver positioning and/or timing information independently from GNSS;
• Act as the backup in the event of a GNSS disruption or outage;
• Provide coverage for the EU European territory including in-land waters;
• Be resilient to GNSS failure modes and vulnerabilities (including GNSS frequency jamming and spoofing or unintentional interference);
• Have a TRL greater than 5 for position/navigation services OR greater than 6 for timing services.
• Provide minimum performance of the alternative PNT service for at least 1 day upon GNSS loss, i.e.: (i) Positioning Accuracy (Horizontal and/or Vertical 95%) < 100 m OR Timing Accuracy to UTC (3 sigma) < 1 microsec and, (ii) Availability > 99%
• If the alternative PNT service provides a timing service, traceability to Universal Time Coordinated (UTC) shall be possible.
KPIs of services had to be assessed after one day, 14 days and 100 days of GNSS service loss. If possible, systems should provide position and/or time information in the environments where GNSS cannot be efficiently delivered. In addition, participants were asked to provide a description of the possibility of deploying the services at a local, regional or continental scale.
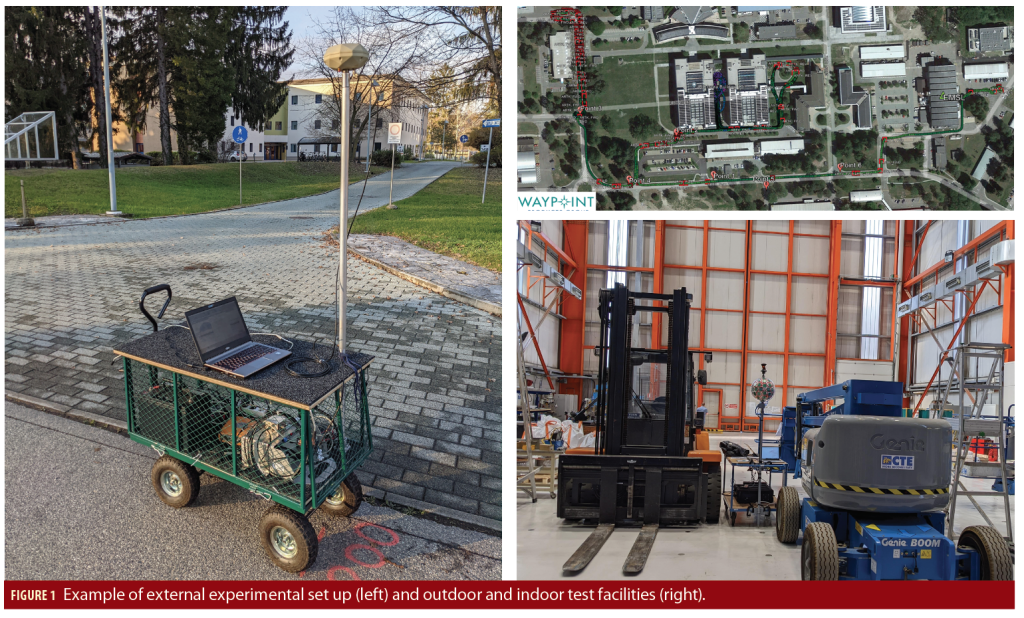
Testing Activities: Set-Up and Achieved Results
Given the diversity of the technologies under investigation, the test plans did not provide a common benchmarking but were agnostic, defined separately for each participant. Both static and kinematic positioning tests were also conducted in varied indoor and outdoor environments. Apart from the tests aimed at a quantitative assessment of the conformity to the requirements set, an additional set of qualitative-based demonstrations of resilience to external threats, remote monitoring and related capacity of the platform were conducted.
The Ispra Site of the JRC, located in Northern Italy, was the main host of the A-PNT demonstrations. It covers more than 170 ha with more than 100 buildings, one to five-floors high, and 36 km of roads, consisting of forest, semi-urban, urban, and rural areas with varied topography, including woodlands. This and the presence of the dedicated labs, including the European Microwave Signature Laboratory (EMSL) [15], makes it ideal for testing and demonstrating PNT technologies in a realistic environment.
Starting in September 2021, almost eight months of testing have been completed, with the A-PNT platforms demonstrating precise and robust timing provision and transfer and positioning services, both indoor and outdoor. Given the tight schedule, some of the demonstrations took place outside of JRC, hosted by the platform providers, as indicated in Table 2.
The JRC was in charge of preparing and running the test campaign, reviewing the projects’ deliverables and test protocols, operating the testing infrastructure within the Ispra site, and managing communication and interactions with each platform provider. The JRC Site Infrastructure Services supported the deployment of the A-PNT demonstrators in the laboratory and in various other locations on the campus. This included installing radio beacons and test equipment both on the roofs of buildings and indoors; interfacing with IT Services to get the required network connectivity; liaising with the Italian Spectrum Regulator to get temporary licenses; installing and operating the test equipment needed to assess the performance of the A-PNT demonstrators; and collecting and processing data using JRC test equipment.
Time scale and reference frame
For the tests conducted on-site, JRC provided static and kinematic references in the European Terrestrial Reference Frame (ETRF) both indoor and outdoor. A grid of permanent points, in the ETRF, were established using GNSS RTK around the campus to support outdoor trials. As part of the process, an indoor reference coordinate system was also established in some of the buildings.
Figure 1 shows an example of an external experimental set up (left) and of the test facilities, both outdoor and indoor (right).
JRC provided a common time reference for all the experiments conducted at the JRC Ispra site. The test rig was set up separately for each platform provider. The time scale used was traceable to UTC(IT) following a calibration made by the Istituto Nazionale di Ricerca Metrologica (INRiM), the Metrology Institute providing the official Italian UTC time scale. This calibration was made using a GNSS timing receiver fed with the 10 MHz and PPS signals from the JRC reference timing rig. This guaranteed the availability of a timing reference, traceable to UTC(IT), with an uncertainty well below 10 nsec.
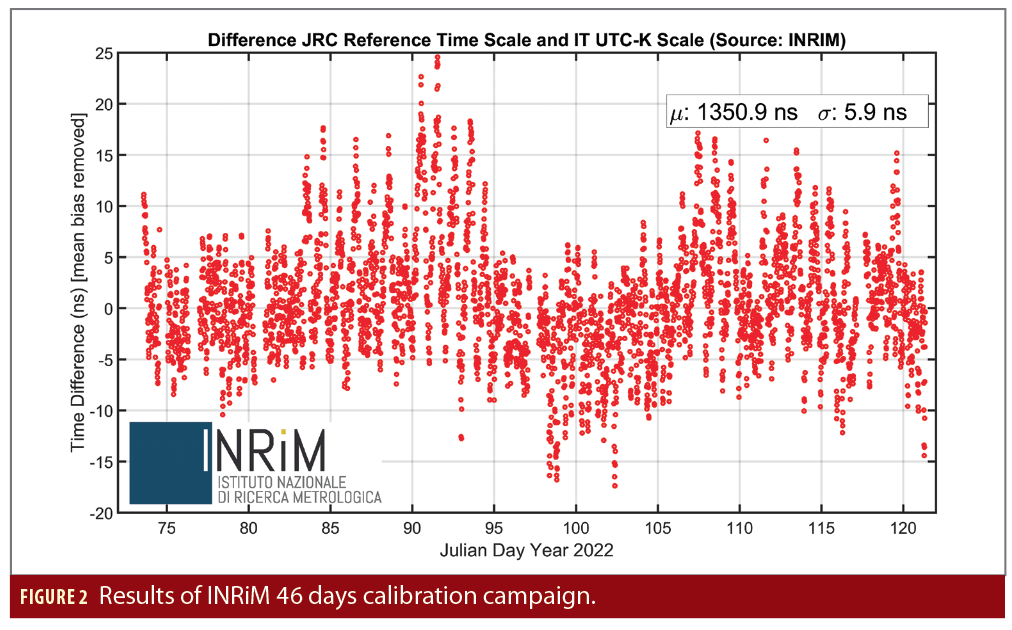
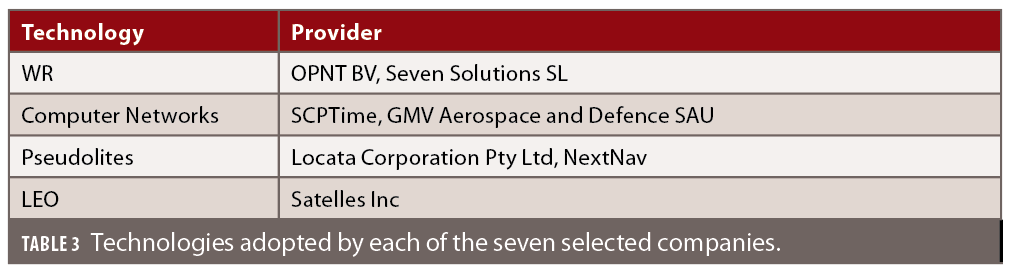
Demonstrated Technologies
The main technologies at the basis of the solutions proposed by the seven selected providers are White Rabbit (WR), computer networks, pseudolites and LEO. The next subsections offer a brief presentation of each, while Table 3 maps each technology with the providers that adopted it.
White Rabbit protocol
The IEEE-1588-2019 High Accuracy (HA) profile, widely known as the White Rabbit protocol, is a time and frequency distribution protocol, developed at the European Council for Nuclear Research (Conseil Européen pour la Recherche Nucléaire, CERN). It combines Precise Time Protocol (PTP) packets with the frequency base of Synchronous Ethernet (SyncE) to provide sub-nanosecond time transfer accuracy over an optical fibre.
To maximize both the availability and continuity of the service, the technologies under test performed best with at least two sources of time (e.g., GNSS, atomic clock, NMI, etc.). As an example, OPNT BV and Seven Solutions SL can seamlessly switch between multiple time sources to ensure continuous transfer with sub-nanosecond accuracy.
Additionally, OPNT demonstrated the technology can monitor and timestamp LTE signals and detect and (if necessary) correct timing errors (drift).
Fixed telecom networks time distribution
Fixed telecom networks use electrical current modulation to distribute data. In the case of the digital transmission, data packages are used to synchronise time, for example using Dynamic synchronous Transfer Mode (DTM). That architecture was standardized by the European Telecommunications Standards Institute (ETSI) in 2001.
The accuracy of such transfer depends on the channel jitter and network asymmetry. The latter is managed by carefully designing monitoring boxes deployment within existing infrastructure. The former is directly related to the intensity of the other, non-related traffic on the same medium. This can be mitigated by increasing the probe packet rate, which requires a guaranteed amount of bandwidth. The paid MPLS network is the critical and sufficient requirement for 200 to 500 ns accuracy.
The density of the boxes also allows for increased resilience. As we need to calibrate each fixed network path, design balances allowed error-budget with the number of nodes installed. Each node also provides monitoring and auto-calibration of overlapping links. Given the cost, the effort to maintain the accuracy might be focused on the main backbones with other connections managed on a ‘best effort’ basis, as long as those connections are not too long (which limits both the number of possible paths and the traffic effect). Results from JRC testing also suggest calibration on a ‘best effort network’ using GNSS as a time source is not enough to maintain reliable service.
The SCPTime demonstrated a proprietary time protocol that additionally provided integrity of the time information transmitted (NTP STS), while DTM time transfer was used by GMV and Locata.
Pseudolites
Pseudolites are a terrestrial positioning technology that use a network of ground-based transmitters providing a robust radio-positioning signal within a specific area. The network needs to be synchroniszd on the nano-second level to provide accurate position and time information.
JRC investigated two different solutions for synchronization: atomic clock oscillators, used by NextNav, and a proprietary frequency alignment for internal synchronisation, adopted by Locata.
Solutions based on pseudolites require large infrastructure investments for a dense network of terrestrial stations. Both participants suggested options to lower deployment costs. NextNav proposed using existing LTE signals, calibrated with its sensors. Locata proposed a sparser network for timing transfer only.
As for the bands allocation, Locata currently uses a Wi-Fi 2.4 GHz frequency while NextNav uses a 920 to 928 MHz frequency band, established in the U.S. for Location and Monitoring Services (LMS). Both companies advocate for the dedicated and protected terrestrial signal band within the EU to increase signal protection.
LEO
LEO systems constituting hundreds or possibly thousands of satellites transmitting from an operational altitude between 400 and 1,500 km. Until recently, this altitude was predominantly used by Earth Observation (EO) and communication satellites (SatCom), while today the potential for LEO-PNT systems is under investigation [3]. LEO offers low latency and high received signal strength (30 dB higher than Medium Earth Orbit, or MEO) at a low transmission power. On the other hand, the orbital positioning closer to the Earth’s surface also has some drawbacks. In particular, the typical satellite footprint (i.e., the ground area that can be covered by the transmitting antennas) is much smaller than MEO satellites (e.g., nine LEOs are required to cover the footprint of one MEO). In addition, the satellite’s relative speed to the ground is much higher, resulting in the need for much larger constellations for full Earth coverage and a higher Doppler rate of the received signals [4,5].
LEO systems are under investigation as potential complementary solutions for classical MEO positioning systems. Currently, LEO orbits are being populated with an increasing number of wide-bandwidth constellations such as SpaceX, OneWeb and IRIS2.
Satelles is a U.S.-based PNT service provider, offering proprietary STL signal transmitted via the Iridium LEO constellation. During JRC testing, they demonstrated timing and position capacity and the possibility of operating both outdoors and indoors. The Satelles infrastructure consists of space (owned by Iridium), ground control (partially shared with Iridium) and user segment. There is no intermediate need for further infrastructure deployment to use the service.
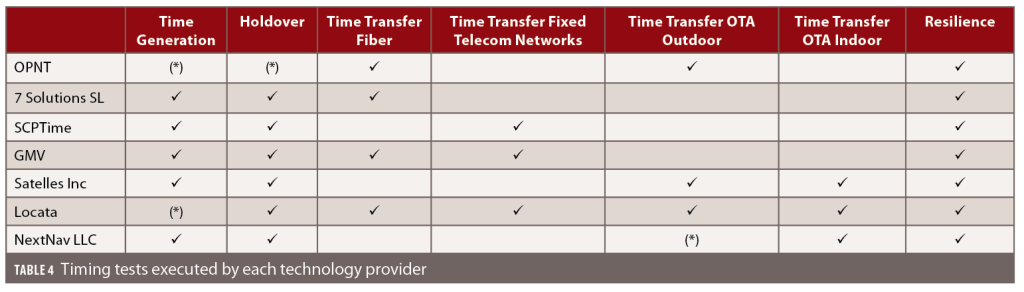
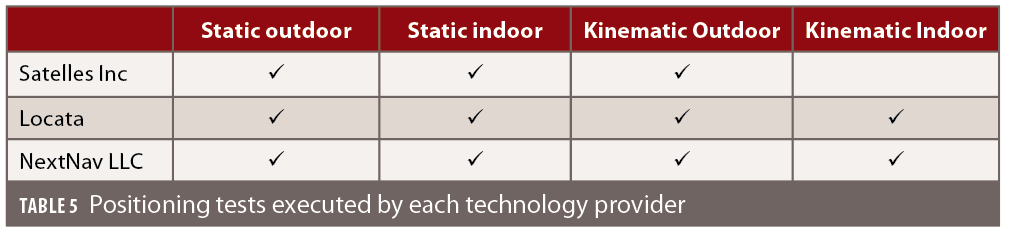
Tests Executed
The test campaign was firstly aimed at understanding the maturity and the readiness of the A-PNT technologies for operational use, and secondly, to assess the performance of the proposed PNT platforms against common baseline KPIs to understand the advantages and limitations of each solution. Given the diverse nature of the technologies presented, the priority was not given to direct comparison, but rather to assess the single solutions. Despite their quantitative nature, the results of the tests, presented in [13], are not strict performance benchmarks, but a qualitative assessment of the technologies. This is because of the discussed agnostic nature of the tests as well as the fact some critical elements (including operation mode and implementation) cannot be captured by KPI metrics and simplifications were made for the sake of the assessment.
This means results should not be used to compare technologies but to understand each technology by itself in isolation. The results of the tests on the single technologies are published in separate publicly available reports [14] and summed up together in [13].
Tables 4 and 5 report, respectively, mapping between each technology provider and the tests executed for timing and positioning. A cell with a tick (V) corresponds to a test carried out, while a cell with an asterisk (*) corresponds to a test that could not be completed because of the unavailability of some test equipment (e.g., a timing source with the required stability could not be made available) or was not required in the context of the proposed test plans.
The main conclusion of the test campaign is that all A-PNT platforms fulfilled the minimum requirements set in the tender. In addition, as far as time generation is concerned, the solutions proposed are a combination of local and remotely located time sources. A-PNT platforms using a local time source were based on a local oscillator with stability comparable to those of Caesium or Passive Hydrogen Masers (PHM) atomic clocks, which was needed to maintain the required time accuracy for up to 100 days in the absence of GNSS. Those using a remotely located time source were based on the time transfer over fiber or over the air from the location of the remote clock (e.g., an NMI distributing the official UTC time scale).
Conclusions and Way Forward
Results show that mature A-PNT solutions exist to provide precise and robust timing and positioning services independently from GNSS. All A-PNT platforms tested fulfilled the minimum requirements set in the tender. Some technologies can complement GNSS by providing services in environments where GNSS is not available, i.e., indoors, but no A-PNT technology can compare to the ubiquity and performance of GNSS services.
The timing technologies include time transfer over long distances through different means, including OTA, fiber and wired channels. Demonstrated time transfers were at the microsecond level, with some of them at the sub-nanosecond level. For time generation, the solutions are a combination of local oscillators and distributing UTC directly from the National Meteorological Institutes, using GNSS-independent time transfers. The position demonstration was conducted by three companies: Satelles, Locata and NextNav. The accuracy of those demonstrations varied from tens of meters up to the centimeter level, also in harsh environments. Tested technologies either require the deployment of terrestrial receivers (beacons, transceivers) or the use of a LEO signal.
The stringent cybersecurity of each A-PNT technology demonstrated during the test campaign is very promising. This includes secure remote access to hardware, secure over the air updates, monitoring and reporting. Hardware also deploys modern programming techniques such as virtualization, allowing for rapid deployment.
The EC is working on multiple solutions to enhance the resilience of EU PNT, such as the evolution of Galileo and EGNOS, the issue of specific regulations, and an upcoming update of the European Radio Navigation Plan. On top of that, some important recommendations have been drawn from the tests campaign. They can be summarized in the following points:
• The test campaign highlighted the important role of the National Measurement Institutes (NMIs) across Europe, as most of the tested technologies work with them directly and indirectly.
• OPNT, Seven Solutions and Locata demonstrated the ability to handle multiple master clock inputs with voting and seamless switchovers.
• Interconnecting as many NMIs as possible, with possible local atomic clocks backups, would result in a very robust and resilient time architecture, independent from GNSS across Europe. The ability to provide resilient and accurate time through the EU communication infrastructure, ideally on the nanosecond level, would also enable robust positioning, using a combination of signals.
• A resilient EU PNT requires a ‘system of system’ approach with a mix of technologies supported by industry standards to ensure the required interoperability. All positioning technologies should operate within the ETRF and all the timing solutions shall be related to the UTC time scale, referenced to an NMI.
• In addition, it is worth mentioning the advantage of having a dedicated spectrum band for terrestrial PNT services, something that is not available in the EU. This alternative already exists in the U.S., with the 902 to 928 MHz frequency band, where a waiver from the US FCC has been granted to provide terrestrial PNT services. This would offer better legal protection against potential sources of radio frequency interference (RFI), but also would allow for an increased transmission power, thus increasing signal robustness and extending the signal range and in-building penetration.
References
(1) European Commission, ‘European Radio Navigation Plan 2023’, 2023, ISBN 978-92-68-00079-3, doi:10.2889/245554. Available at: https://joint-research-centre.ec.europa.eu/document/download/ea1f597d-24f6-493b-a209-06cc9245dcd6_en?filename=ERNP%202023.pdf
(2) John A. Volpe National Transportation Systems Center (U.S.) “Vulnerability assessment of the transportation infrastructure relying on global positioning system”, 2001
(3) F. S. Prol et al., “Position, Navigation, and Timing (PNT) Through Low Earth Orbit (LEO) Satellites: A Survey on Current Status, Challenges, and Opportunities,” in IEEE Access, vol. 10, pp. 83971-84002, 2022, doi: 10.1109/ACCESS.2022.3194050.
(4) R. Morales-Ferre, E. S. Lohan, G. Falco and E. Falletti, “GDOP-based analysis of suitability of LEO constellations for future satellite-based positioning,” 2020 IEEE International Conference on Wireless for Space and Extreme Environments (WiSEE), Vicenza, Italy, 2020, pp. 147-152, doi: 10.1109/WiSEE44079.2020.9262624.
(5) R. Morales Ferre, J. Praks, G. Seco-Granados and E. S. Lohan, “A Feasibility Study for Signal-in-Space Design for LEO-PNT Solutions With Miniaturized Satellites,” in IEEE Journal on Miniaturization for Air and Space Systems, vol. 3, no. 4, pp. 171-183, Dec. 2022, doi: 10.1109/JMASS.2022.3206023.
(6) H. Wymeersch, G. Seco-Granados, G. Destino, D. Dardari, and F. Tufvesson, “5G mm-Wave positioning for vehicular networks,” IEEE Wireless Communications MagazineVol. 24, no. 6, pp. 80-86, 2017.
(7) J. A. del Peral-Rosado, R. Raulefs, J. A. López-Salcedo, G. Seco-Granados, “Survey of cellular mobile radio localization methods: From 1G to 5G,“ IEEE Commun. Surv. Tutor., Vol. 20, pp. 1124-1148, 2018.
(8) R. Keating, M. Säily, J. Hulkkonen and J. Karjalainen, “Overview of Positioning in 5G New Radio,” 2019 16th International Symposium on Wireless Communication Systems (ISWCS), Oulu, Finland, 2019, pp. 320-324, doi: 10.1109/ISWCS.2019.8877160.
(9) Enhanced Loran (eLoran) Definition Document. International Loran Association, version 0.1. 12 January 2007
(10) G. W. Johnson, P. F. Swaszek, R. J. Hartnett, R. Shalaev and M. Wiggins, “An Evaluation of eLoran as a Backup to GPS,” 2007 IEEE Conference on Technologies for Homeland Security, Woburn, MA, USA, 2007, pp. 95-100, doi: 10.1109/THS.2007.370027.
(11) Lukas Hosch, Filippo Giacomo Rizzi, Lars Grundhöfer, Ralf Ziebolda and Daniel Medina, “Backing-Up GNSS with R-Mode: Positioning Performance for Recursive Estimators,” ICL-GNSS 2022 WiP, June 07–09, 2022, Tampere, Finland
(12) Alternative Position, Navigation and Timing (PNT) Services. 2020/S 208-506573. Contract notice. 26/10/2020. Available at: https://ted.europa.eu/udl?uri=TED:NOTICE:506573-2020:DATA:EN:HTML
(13) Bonenberg, L., Motella, B. and Fortuny Guasch, J., Assessing Alternative Positioning, Navigation and Timing Technologies for Potential Deployment in the EU, EUR 31450 EN, Publications Office of the European Union, Luxembourg, 2023, ISBN 978-92-68-01163-8, doi:10.2760/596229, JRC132737. Available at: https://publications.jrc.ec.europa.eu/repository/handle/JRC132737
(14) https://joint-research-centre.ec.europa.eu/scientific-activities-z/alternative-pnt_en#assessing-alternative-positioning-navigation-and-timing-technologies-for-potential-deployment-in-the-eu
(15) Cucchi, L., Gioia, C., Susi, M., Damy, S., Bonenberg, L., Boniface, K., Basso, M., Sgammini, M., Paonni, M. and Fortuny Guasch, J., JRC Testing and Demonstration Hub for the EU GNSS Programmes, EUR 30747 EN, Publications Office of the European Union, Luxembourg, 2021, ISBN 978-92-76-39185-2, doi:10.2838/93444, JRC125180. Available at: https://publications.jrc.ec.europa.eu/repository/bitstream/JRC125180/jrc_gnss_testing-capabilities-inventory_clean_pubsy_1_1.pdf
1DA/FCC #: DA-20-755, Docket No: 12-202, FCC Record Citation: 35 FCC Rcd 7136 (9), FCC Record: https://docs.fcc.gov/public/attachments/DA-20-755A1_Rcd.pdf
Authors
Matteo Paonni is Deputy Head of the Technologies for Space, Security and Connectivity Unit, within the Directorate for Space, Security and Migration at Joint Research Centre of the European Commission in Ispra, Italy. Under his position, Matteo coordinates the scientific and policy support on the EU GNSS Programmes. His main technical background is on GNSS signal design and optimization, GNSS security, compatibility and signal processing. From 2007 to 2013 Matteo was a research associate at the University of the Federal Armed Forces in Munich, Germany.
Beatrice Motella is Project Officer at the Joint Research Centre of the European Commission, within the Technologies for Space, Security and Connectivity Unit. She holds a Ph.D. in Electronics and Communications Engineering obtained from Politecnico di Torino. Her activities cover different aspects of radio navigation, with a major focus on Galileo signal authentication and signal processing.
Lukasz Bonenberg
is a Space Programmes Policy and Scientific Officer at the Joint Research Centre (JRC) of the European Commission in Ispra, Italy. His previous employment included the University of Nottingham (from which he received a Ph.D. in Space Geodesy) and the private sector. His recent work focuses on the analysis of alternative PNT technologies and on the preparation of the European Radionavigation Plan.
Joaquim Fortuny-Guasch received an engineering degree in telecommunications from the Technical University of Catalonia (UPC), Barcelona, Spain, and a Dr.-Ing. degree in electrical engineering from the Universität Karlsruhe (TH), Karlsruhe, Germany. Since 1993, he has worked for the Joint Research Centre (JRC) of the European Commission, Ispra, Italy, as senior scientific officer.
Ignacio Alcantarilla Medina holds a degree in Aeronautics from the Polytechnic University of Madrid (UPM), Spain. He worked for 12 years in the Space industry and in 2012 joined the European Commission, where he works in the EU Space Programme. He has recently coordinated the European Radio Navigation Plan 2023.