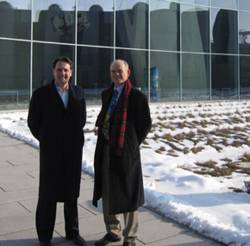
We appear at the gatehouse beside the public entrance marked “DLR” a few minutes early for our appointment — as befits a visit to this sprawling display of German aerospace expertise that is itself usually a good deal ahead of the times. It’s the day after the close of this year’s Munich Satellite Navigation Summit, and we have taken the S-Bahn down from the city.
We appear at the gatehouse beside the public entrance marked “DLR” a few minutes early for our appointment — as befits a visit to this sprawling display of German aerospace expertise that is itself usually a good deal ahead of the times. It’s the day after the close of this year’s Munich Satellite Navigation Summit, and we have taken the S-Bahn down from the city.
The inevitable paperwork ensues, and we surrender a passport as surety of our good behavior during our stay at the Oberpfaffenhofen facility 25 kilometers west of Munich. Over the next five hours we will be offered a sampler plate of the leading-edge GNSS activities underway here, capped off with visits to the new Galileo Control Center and an excursion to the agency’s 30-meter high-gain antenna near Weilheim.
Known by its German name Deutsches Zentrum für Luft- und Raumfahrt and translated more succinctly as the German Aerospace Center, the DLR serves as Germany’s space agency with around 6,500 people working in 29 scientific institutes at 13 locations. With approximately 1,500 people and eight institutes, the Space Research Center Oberpfaffenhofen is one of the largest DLR sites and blends a distinct mix of research, space operations, and industry-sponsored commercial development.
Such a presence naturally acts as a magnet for related initiatives, such as the Anwendungszentrum Oberpfaffenhofen — the Application Center for Satellite Navigation. We have already spent the morning at the Anwendungszentrum negotiating a role for Inside GNSS as global media partner for the European Satellite Navigation Competition (ESNC) and, together with NovAtel, Inc., the sponsors of a new ESNC regional competition for the United States. (See article on page 38.) Not surprisingly, DLR is one of the special topic sponsoring partners for the ESNC.
At the aerospace center entrance, we are taken in hand by Steffen Thölert, one of the many DLR researchers whom we will meet today. He delivers us to our host, Christoph Günther, director of the Institute of Communications and Navigation, that serves as the focal point for most of the GNSS-related activities at Oberpfaffenhofen — particularly in the ICN’s Department of Navigation headed by Michael Meurer, who has arranged our schedule at DLR.
The Oberpfaffenhofen site has been a center of aviation activities since the 1930s, although the DLR presence has been a more recent development. The forerunner to the ICN, Institute for Satellite Electronics, was established in 1966 by Philip Hartl, then-director of the Oberpfaffenhofen center. (Hartl was a coauthor for a three-part Inside GNSS series in 2007, “Envisioning a Future: GNSS System of Systems.”)
In 1982, four years after the launch of the first GPS satellite, Oberpfaffenhofen engineers built what is perhaps Europe’s first GPS receiver, Christoph told us.
In addition to satellite navigation R&D, the center’s main fields of activity at Oberpfaffenhofen include participating in space missions, climate research, research and development in the field of Earth observation, and advanced robotics development. The Spaceflight Operations and Astronaut Training Center and the DLR Gesellschaft für Raumfahrtanwendungen (Space Applications Company), a German Aerospace Center entity spun off recently as a separate company.
A few weeks before our visit, Oberpfaffenhofen learned that it had received DLR’s designation as a “Center of Excellence for Satellite Navigation,” a three-year (2010–2012) award that brings additional resources to the institute’s efforts in this area. Meurer will serve as coordinating director for the center of excellence.
GBAS Demonstrator: Safety-Critical Navigation
Following his overview of the ICN’s activities, Günther led us to a nearby office housing a multimodular workstation where Boubeker Belabbas introduced DLR’s ground-based augmentation system (GBAS) demonstrator for precision approach and automatic landing of aircraft.
The institute is part of an international effort to create navigation subsystems that rely on GBAS to provide approach and landing in all weather conditions up to the so-called Category III (CAT-III) level with zero visibility. By means of an Internet TCP/IP link, the demonstrator workstation can monitor and control flight tests conducted from DLR’s research airport hundreds of miles away in Braunschweig.
On the screen we follow a recorded playback of a CAT I flight demonstration conducted successfully last year with a Dornier 228 aircraft. (DLR researchers will soon have an Airbus A320 with which to develop and test their CAT III system.)
Display screens showed such variables as number of satellites tracked, ionospheric conditions affecting GNSS signal propagation, real-time estimates of position accuracy, and so forth. One screen displayed the aircraft icon surrounded by a three-dimensional error ellipse reflecting its position and another graphical bubble representing the safety zone given by the protection levels. The position error ellipse needs to stay inside the protection level zone in order to avoid hazardously misleading information and to meet safety-of-life requirements.
The Braunschweig airport is equipped with three dual-frequency (L1/L2) GNSS receivers providing real-time data to the GBAS central processing facility, which calculates differential corrections and monitors signal integrity. Corrections and integrity information are included in the standard message types that are then broadcast to the aircraft during its approach phase.
On board the aircraft, a multi-mode receiver applies pseudorange corrections and range-rate corrections received from the ground subsystem and computes a position, velocity, and time (PVT) solution. At the same time, integrity information is processed onboard using the error bounds information provided by the ground processing facility. The output of the airborne processing as well as airborne raw data is broadcast via the TCP/IP link back to the GBAS demonstrator workstation.
The modular and highly flexible structure of the unique GBAS demonstrator allows researchers to easily integrate and test new software processors in the system as well as to implement and test improved signal quality monitoring, multipath, and interference mitigation/monitoring algorithms.
Belabbas explained how the aircraft navigation and flight control functions stem from two different technology paths — based on instrument landing system (ILS) values and manual control techniques, respectively — that must be merged in GNSS-aided autolanding.
Ultimately the GBAS demonstrator will be used as the development platform for future concepts, including multi-constellation, multi-frequency GBAS to enable full CAT III capabilities, carrier phase¬–based solutions including integrity monitoring to enable auto-taxiing and full gate-to-gate navigation, inertial hybridization techniques to improve robustness, and coupling of the autopilot and GBAS system to explore a total performance concept.
Multisensor Navigation: NaviBoots and Quadrotors
We bid farewell to the GBAS project and head down to the office of Patrick Robertson where we discover some of the coolest toys in the DLR toolbox.
Readers can learn much more about the results of Robertson and his colleagues’ work in the area of simultaneous localization and mapping in the article, “SLAM Dance: Inertial-Based Joint Mapping and Positioning for Pedestrian Navigation,” in this issue. But that seriously technical exposition doesn’t quite convey the kind of fun that goes along with it.
The FootSLAM concept builds on GPS/inertial sensors that log position data as the system moves through a building mounted on a person. As the sensor platform enters a building, the GPS receiver provides a point of reference in an absolute coordinate system, from which the inertial measurement unit (IMU) dead-reckons subsequent positions.
Whenever the person wearing the sensor platform returns to an earlier point in the traverse through the building, the SLAM algorithms begin to construct an internal representation of the interior corridors, doors, walls, and large objects that are circumnavigated such as tables.
Robertson’s team has developed a foot-mounted platform for the sensors called the Navishoe, admittedly inspired by the similarly named NavShoe trademarked by InterSense Inc. (Billerica, Massachusetts, USA). (The DLR’s Navishoe actually looks more like a hiking boot, but NaviBoot might cause some confusion because “Boot” is German for “boat.”)
Last year InterSense announced its new inertial product, the NavChip, a micro-electromechanical system (MEMS) IMU, which it is fitting into the NavShoe. Robertson and his colleagues have worked with the NavChip, but their current platform uses the MTx orientation and motion tracker from Xsens Technologies B.V. (Enschede, The Netherlands).
DLR’s SLAM researchers are now trying to develop an autonomous aerial mapping platform using quadrotors — lightweight craft with four propellers on which they intend to mount IMUs, GPS, miniature cameras, and laser scanners that can fly outdoors or indoors, avoiding obstacles as they map an area. The researchers have fondly named two of the micro aerial vehicles “Wilbur” and “Orville” after the American airplane inventors, the Wright brothers.
With a little urging, Robertson and his colleagues escort us downstairs to the “holodeck,” an indoor flight testing space with an array of eight high-frequency infrared strobe/camera units. There, two young associates, who look like they’d know their way around a Sony PlayStation, demonstrate remote control of a semi-autonomous quadrotor.
The tracking system determines the quadrotor’s exact position and attitude in the lab’s reference frame, explains Michael Angermann, another member on the DLR SLAM team. The researchers have modified the software of a commercial product, Vicon Tracker, to enable the system to log, stream, and visualize the quadrotor’s position and attitude — typically by projecting the visualization onto one of the lab walls.
Researchers can attach small reflecting spheres to a rigid body — in this case, the white polygonal balls visible on the quadrotors in the accompanying photos — and put this body into the field of view of the cameras. The system sees the spheres with multiple cameras at the same time.
With the cameras’ positions and directions known, Robertson, Angermann, Mohammed Khider, and their teammates can compute the position of each sphere — each frame a camera captures essentially gives an “azimuth” and “elevation” angle under which the cameras sees a sphere.
“One can then tell the system to consider a set of spheres to belong to one rigid object and give this body a name, say “Charles,” says Angermann. “We can then define an origin and a rotation of this object and, voila, the system shows the “icon” for Charles on the system.”
The researchers have developed software that enables the micro aerial vehicle to maintain its horizontal stability automatically. Still ahead are autonomous vertical stability and navigation.
Ultimately, the team envisions a world in which travelers, upon arrival at a new destination, can download cached maps to their mobile phones — already typically equipped with cameras and MEMS inertial devices — and use these to navigate through the unfamiliar locale.
These maps may one day be derived from user-generated sensor data collected on cell phones, which are commonly equipped with cameras and inertial devices (such as accelerometers). The information may be gathered by individuals who themselves have no immediate need for positioning or navigation and made available for those who do.
GALANT: A Robust GPS/Galileo Multi-Antenna Receiver
We make our way out into the brisk March air and over to the basement of another building, where Manuel Cuntz and Andriy Konovaltsev are waiting to show us the latest incarnation of GALANT, a Galileo/GPS receiver demonstrator with a multiple-element antenna array.
A cable runs from the room outside to an antenna tripod about 10 meters away from the building in an open area from which satellites can be tracked.
The combination of an adaptive, digitally steerable antenna and a real-time multichannel L1/E1/E5a receiver realized as a field programmable gate array (FPGA) is designed to enable DLR researchers to test interference detection and mitigation techniques needed for safety-of-life applications, particularly in civil aviation. The The raw data coming from the receiver RF front-ends can also be recorded and processed offline in a MATLAB-based software receiver.
Currently, the antenna array is a 2×2 configuration, but the final architecture will use a 16-element (4×4) design. The receiver processes four independent digital data streams passing through four RFICs from the four antenna elements.
GALANT uses digital beam-forming to detect the digital data streams and high-resolution algorithms to provide directions of arrival (DOA) estimations of GNSS satellite signals with an accuracy of three degrees max deviation.
Moreover, by beam-forming for each satellite, optimized antenna patterns are digitally steered towards the useful satellite signals. This selective reception, along with algorithms that enable nulling and the suppression of target sidelobes, allows the nearly complete elimination of detrimental effects of multipath, unintended interferers and jammers on the navigation receiver performance. In turn, this detection and mitigation of interference increases the availability and integrity of positioning.
As with offices and work areas we have visited so far, the GALANT lab is stacked with GNSS and test equipment: the DLR Master (a special setup of several Spirent GSS7790 simulators suited for testing of multi-antenna GNSS receivers), Topcon, Novatel, Javad, Septentrio, and other receivers, as well as a computer workstation with multiple windows analyzing various elements of the real-time signal processing.
Galileo Control Center
Standing out among the mostly utilitarian structures on the Oberpfaffenhofen campus is a concave glass-fronted building with a notably different look and feel to it. This structure houses the Galileo Control Center (GCC), which opened December 8, 2008, with a multi-national, multi-company European operations team.
Construction of the new control centre, with more than 3,000 square meters of floor space, was completed in just two years. (A time-lapse video of the construction makes the work appear to fly by even faster.) The fully equipped building cost about €100 million with architectural services provided by Schultes Frank Architekten, the same Berlin firm that designed the Federal Chancellery building.
A full-scale model of a Galileo in-orbit validation (IOV) satellite hangs in the lobby of the control center, covered in black insulating foil — a surprising feature after more than 10 years of artist’s renderings of Galileo spacecraft that portray them wrapped with bright gold foil.
By the time the Galileo satellite navigation system is fully operational, up to 100 engineers and scientists will be working at over 30 control consoles in the control center. With only two Galileo spacecraft — GIOVE-A and -B — in orbit, however, the staffing level is currently about 30, we are told by the center’s managing director, Walter Päffgen. A successful Galileo system validation test was conducted at the GCC last November.
In 2005, Päffgen became head of the Galileo Operations department in the DLR’s German Space Operations Center also located at DLR-Oberpfaffenhofen. DLR spun that department off in 2008 as the DLR Gesellschaft für Raumfahrtanwendungen (Space Applications Company), with Päffgen moving to the new organization as technical managing director. DLR GfR has a contract with the European Space Agency to support IOV operations.
The Galileo Control Center in Oberpfaffenhofen is part of the core system operations, specifically the satellite control segment, and will share responsibilities with a counterpart facility in Fucino, Italy. The center tracks and controls the satellites through a globally distributed ground station network.
Galileo system time will be generated on the ground with the aid of atomic clocks installed in the control centers and transmitted to the satellites via the ground network. Both the cesium and hydrogen maser frequency standards at the GCC are being supplied by Symmetricom, a U.S. company based in San Jose, California.
DLR GfR and Italy’s Telespazio have formed spaceopal GmbH and lead an industrial team that is bidding for the FOC Galileo operations work package, which ESA expects to award this summer.
Weilheim: What a Dish!
Up close and personal, the curved aluminum antenna dish at Weilheim — painted white and aimed vaguely skyward, with a big Krupp corporate logo on the front — looked much bigger than 30 meters across. And when we climbed up the steel stairs to the platform half-way up the dish, the ground looked a lot further away than 20 meters.
And that was before Peter Speck, overseeing the DLR facility this late winter afternoon, flipped the switch and set the antenna in motion — rotating 240 degrees in each direction horizontally, 90 degrees in the vertical.
The motor driving the dish can move it about 1.5 degrees per second, our hosts told us. But they usually keep it under half a degree per second. No autobahn excesses here. Still, it felt a bit like the slow-motion equivalent of a Tilt-a-Whirl. All in the pursuit of scientific research, of course.
After about four hours of shuttling us between buildings to view projects at Oberpfaffenhofen, Thölert and fellow DLR researcher Johann Furthner had packed us into a car and driven about 35 kilometers southwest to the space agency’s ground station at Weilheim. En route we had a chance to admire the multi-million-euro residences along the Ammersee, an area that became fashionable when the railroad from Munich first reached the lake region at the turn of the 20th century.
Built in 1972, one of the antenna’s first missions was to operate and control the Helios I and II probes in a joint U.S.–German mission that studied the solar wind from a path that stretched inside Mercury’s orbit (at perihelion). The Helios instrumentation is still bolted into place inside the cabin where the Galileo signal monitoring package now resides, but only the latter is operational.
Inside the cramped cabin accompanying the 30-meter dish, DLR’s investment in technology is once again visible. In a rack alongside the tube channeling the antenna feed, shelves hold Agilent and Rohde & Schwarz vector signal analyzers, a Rohde & Schwarz signal generator, a network analyzer, and several other boxes filled with electronics — all part of the instrument suite with which DLR monitors and verifies Galileo and other GNSS signals.
DLR scientists at Oberpfaffenhofen can remotely track and monitor signals from GIOVE-A and –B using the big dish at Weilheim, which also monitors signals of other GNSS satellites. It will also play a key role in operating the in-orbit validation (IOV) and succeeding Galileo satellites to be launched from Kourou, French Guiana, beginning early next year.
It’s a data-intensive business to record the transmissions received at the site: 30 milliseconds of in phase and quadrature data from a Galileo signal take up to 20 megabytes of disk space. A whole pass of the satellite over Weilheim requires data from several snapshots of that kind, which take up well more than several gigabytes of disk space, Furthner says, too much to try relay to Oberpfaffenhofen. Indeed, excursions to swap out storage media measured in terabytes represents one of the main reasons for trips to Weilheim.
The aerospace center researchers have an enviable access to the antenna resource, because the agency’s Galileo/GNSS activities currently represent the only regular long-term use of the 30-meter Weilheim dish.
But That’s Not All
Despite what seemed like a very full schedule, our tour only scratched the surface of the GNSS-related activities at the German Aerospace Center. Some of the others include:
- The Car 2 Car Wireless Communications cooperative driving initiative that fuses GNSS, INS, radar, and LiDAR
- GBAS for maritime use — the world’s first Carrier Phase IALA DGNSS Demonstration System to aid berthing of ships
- the world’s first testbed for navigation using LTE or long term evolution (4G, fourth generation mobile telephony), employed in the WHERE and GRAMMAR projects on hybrid navigation (mobile radio/GNSS) for which DLR provides leadership
- ionospheric monitoring, modeling, and prediction within the Space Weather Application Center Ionosphere (SWACI) using the EVnet (Experimentation and Verification Network) of high-precision GNSS receivers linked via Internet connections to a central processing and control center;
- the Galileo Evolution C-band signal design project (described in a Working Papers series in the May/June and July/August 2009 issues of Inside GNSS);
- railway collision avoidance and disaster management projects and many others.
And that’s just the navigation side of ICN; communications researchers have their own portfolio of leading-edge research projects such as high-rate data transfer (including optical transmission methods) from deep-space missions, earth-orbiting satellites, and aeronautical platforms.
But let’s save that for another fine day.