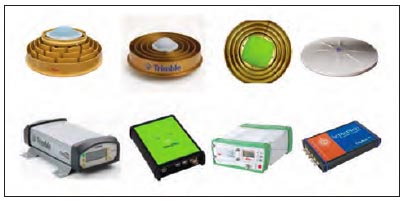
Over the past decade, the world of global positioning has experienced dramatic changes. Starting from just a single constellation (GPS), a set of six global or regional navigation satellite systems — with the addition of GLONASS, BeiDou, Galileo, Quasi-Zenith Satellite System (QZSS) and the Indian Regional Navigation Satellite Systems (IRNSS) — has emerged that are already offering, or at least preparing, a space-based position, navigation, and timing (PNT) service. These independent services are further complemented by a variety of satellite-based augmentation systems (SBASs) to increase the availability, accuracy, and reliability of PNT for safety-critical applications.
The potential merits of the new navigation satellite systems have long been praised and often been used to justify the need for their build-up. Leaving aside the political arguments for an independent national PNT infrastructure, multi-constellation systems can indeed offer numerous advantages over stand-alone GPS navigation and the use of its legacy ranging signals. New signal structures will not only provide a greater robustness against interference and multipath but also enable more robust tracking at low signal levels.
The availability of unencrypted signals on three frequencies enables new approaches to ambiguity resolution in carrier-phase–based relative positioning and may also contribute to the analysis of higher-order ionospheric path delays. Last, but not least, the simple increase in the number of available satellites not only enhances navigation applications, but also offer an increased number of signals for space weather applications that employ occultation techniques and ray tracing of the neutral atmosphere and the ionosphere.
Given the remarkable contributions to science that GPS continues to make in geodesy, remote sensing, space, and fundamental physics, similar and even larger benefits are commonly expected from the new space-based PNT systems. Many of the cited applications make direct or indirect use of the International GNSS Service (IGS), which has, over many years, set the gold standard for high-precision GPS and GLONASS measurement modeling and analysis.
The IGS is a volunteer organization of more than 200 individual agencies and institutions that maintain a global network of monitoring stations and a long-term tracking data archive as well as products derived from the analysis of these measurements.
With the advent of modernized GPS signals and the rise of numerous new GNSS such as BeiDou, Galileo, QZSS, and IRNSS as well as new augmentation systems such as Russia’s System of Differential Correction and Monitoring (SCDM) and India’s GPS Aided GEO Augmented Navigation (GAGAN) system, the IGS is fully committed to expand to a true multi-GNSS service.
To pave the way for future provision of high-quality data and products for all constellations, the IGS has initiated the Multi-GNSS Experiment (MGEX) under coordination of its Multi-GNSS Working Group. MGEX serves as a framework for increasing the overall awareness of multi-GNSS within the scientific and engineering communities, as well as to familiarize IGS participants and users with the new navigation satellite systems.
This article will provide a brief overview of new and modernized GNSS constellations and their transmitted signals as available in fall 2013. Thereafter, it describes the MGEX network, which the IGS has established for multi-GNSS tracking and is operated in parallel to the legacy network in use for GPS and GLONASS.
A subsequent section presents initial MGEX data products, assesses their achieved performance, and discusses relevant processing standards. We conclude with a discussion of necessary steps and actions required in order to fully incorporate new constellations and signals into the IGS service portfolio.
Navigation Satellite Systems Status
Table 1 summarizes current space-based PNT systems and operational satellites as derived from the publication by R. Langley cited in the Additional Resources section near the end of this article. With the most recent launch of IRNSS-1A, a total of six navigation satellite systems have so far become available. Among these, the legacy systems GPS and GLONASS have long achieved their full operational capability and provide navigation signals on at least two frequencies (L1, L2) that can be accessed by civil users.
The latest generations of GPS IIF and GLONASS-K satellites have added a third frequency (L5 and L3, respectively), but these signals remain limited to a very small number of spacecraft. In June 2013, the GPS Directorate started an initial test campaign with live broadcasts of the new L2C and L5 CNAV navigation messages.
These offer enhanced navigation information and improved positioning capabilities. The message-populated broadcast is projected to begin April 2014. Users should expect initial CNAV signal accuracy to be less than the legacy signals. Upon full implementation in December 2014, CNAV signal accuracy should meet or exceed the legacy signals.
In addition to GPS and GLONASS, the BeiDou system now offers a standalone navigation service for the China mainland and Asia-Pacific area, with a global service expected to be available by about 2020. Even though the BeiDou Open Service Interface Control Document (ICD) only covers the B1 and B2 Open Service signals at present, signals on up to three BeiDou frequencies can in fact be tracked by a variety of multi-GNSS receivers. BeiDou is thus the first constellation enabling a systematic assessment of triple-frequency positioning techniques.
Galileo presently has four in-orbit validation (IOV) satellites in operation. These satellites support early testing and experimentation but have not yet been declared healthy. An initial operational service with global coverage is targeted for a few years from now. Although access to the Galileo E6 signals is still not fully defined, users can freely access signals with advanced multi-path performance in the E1 and E5a/E5b bands.
As a unique feature, the Galileo satellites are equipped with passive hydrogen masers. These offer exceptional clock stability with many potential benefits for real-time navigation, precise positioning, and science applications.
Japan has validated the concept of QZSS with its “Michibiki” satellite for more than two years. A fully operational QZSS comprising at least three satellites in inclined geosynchronous orbit (IGSO) and one in geostationary orbit (GEO), is envisaged within the present decade. QZSS supports a unique portfolio of navigation signals on four distinct frequency bands and offers various types of correction data for medium and high-accuracy users.
Finally, India launched a first satellite, IRNSS-1A, in July 2013, which is currently undergoing testing. Four IGSO and two GEO satellites will ultimately comprise IRNSS. IRNSS-1A is transmitting signals in both the L5 and S bands, but common GNSS receivers cannot presently track these signals due to the lack of information about the L5 ranging codes employed and the unique choice of the second signal frequency. It is also presently unclear whether addition of an L1 signal is being considered for upcoming IRNSS satellites to improve interoperability with existing systems.
The six navigation satellite systems that we have described here are complemented by a total of 13 SBAS satellites in geostationary orbit. While not in the immediate focus of the IGS and the scientific community, a growing number of SBAS satellites already offer dual-frequency (L1/L5) ranging signals that can be tracked by modern GNSS receivers and may become of interest for future precise positioning applications.
An up-to-date overview of the GNSS system status with focus on new constellations is maintained at the website of the IGS Multi-GNSS project (listed in Additional Resources) along with supporting information for the data processing.
The IGS Multi-GNSS Network
As a backbone of the MGEX project, a new network of multi-GNSS monitoring stations has been deployed around the globe in parallel to the legacy IGS network for GPS and GLONASS. Building on contributions from various national agencies, universities, and other volunteer institutions, the MGEX network had grown to almost 90 stations by September 2013 (See Figure 1).
MGEX largely draws on the resources of institutions that have modernized their legacy GNSS monitoring networks in recent years or established new multi-GNSS–capable monitoring stations. Overall, about two-thirds of all MGEX stations are contributed by France’s Centre National d’Etudes Spatiales (CNES) and the Deutsches GeoForschungsZentrum (GFZ) as well as the Deutsches Zentrum für Luft- und Raumfahrt (DLR), the European Space Agency (ESA), and the Bundesamt für Kartographie und Geodäsie (BKG). The MGEX website provides an up-to-date map and list of all MGEX stations along with links to station-specific sitelog files.
As a minimum, all MGEX stations support tracking of GPS as well as one of the new BeiDou, Galileo, or QZSS constellations. While not a prerequisite, GLONASS is likewise supported by the majority of stations and a large fraction also offers L1 or L1/L5 SBAS tracking. However, no stations with IRNSS tracking capability have, so far, become available for the MGEX project due to the lacking signal specification and the very early project status.
MGEX builds on a highly heterogeneous network comprising a wide range of end user equipment. The most widely used receiver types are listed in the Manufacturers section near the end of this article. Most sites employ choke ring antennas but survey-grade antennas with conventional ground-planes are likewise employed at many stations.
Even though the variety of employed receivers and antennas poses a significant challenge for consistent data processing, this variety is, at the same time, a valuable asset. The diversity of available tracking techniques and types of data employed by the various receivers contributes to a thorough understanding and assessment of new navigation signals. Also, the cross-comparison of different types of equipment contributes to continued evolution and improvement of GNSS receiver design by the manufacturers.
Six MGEX sites presently host multiple receivers of different types connected to a common antenna (Table 2 in Manufacturers section). This enables a direct comparison of the tracking behavior and assists the assessment of receiver-specific differential code and carrier phase biases for individual GNSS signals.
Data centers at the NASA Crustal Dynamics Data Information System (CDDIS), the French Institut Géographique National (IGN), and BKG archive and distribute the observation data and broadcast ephemerides collected by the MGEX network. To facilitate these activities, the Receiver Independent Exchange Format (RINEX 3) has consistently been adopted throughout the MGEX project. Implementation of the latest version 3.02 is in progress, but legacy DOS-style (8+3) filenames are currently retained for compatibility with existing processing infrastructure.
Introduction of new filenames with extended information fields as foreseen in the new RINEX 3.02 standard is planned at a later stage in coordination with MGEX users and the IGS Infrastructure Committee. As a minimum, daily RINEX observation files at a 30-second sampling rate are provided for all stations, but hourly and/or 15-minute high-rate observations files are likewise offered for selected sites through the individual data centers. Links to the MGEX data archives can again be found at the MGEX website.
In addition to offline data, a large subset of stations also provides real-time data streams with multi-GNSS observations to the MGEX project (Figure 2). BKG, Frankfurt, hosts a dedicated online caster for the MGEX project, where interested users can presently access data streams from roughly 70 stations following a free registration. The Networked Transport of RTCM via Internet Protocol (NTRIP) protocol and the RTCM3-MSM Multi-Signal Message format have been adopted for the MGEX project to ensure a consistent interface irrespective of the receivers used and their native binary data formats.
Other than legacy RTCM 3.1 messages, the new MSM messages are designed to handle all constellations, signals, and observation types so as to ensure full compatibility with the information content of RINEX observation files. Following release of the new RTCM 3.2 standard, major receiver manufacturers are preparing implementation of MSM support, but MSM-capable firmware versions have not yet been publicly released.
As a substitute BKG and NRCan have started to generate prototype MSM data streams for the MGEX project by converting native data formats in real-time. The resulting streams are made available at the BKG MGEX caster and facilitate early familiarization and utilization of this format.
Precise Orbit and Clock Products
As a first step towards the incorporation of new constellations into an IGS multi-GNSS service, various analysis centers compute precise orbit and clock products for Galileo and QZSS based on observations of the MGEX network and, optionally, other proprietary stations. The data are publicly available for interested users at the MGEX product archive maintained by the CDDIS. Similar efforts are expected in the near future for BeiDou.
Galileo. Technische Universität München (TUM) and CNES/Collecte Localisation Satellites (CLS) routinely provide orbit and clock products for the four Galileo IOV satellites (PRN E11, E12, E19, and E20) with latencies of three to six days. These are complemented by various batches of reprocessed ephemeris contributed by the Center for Orbit Determination in Europe (CODE) and GFZ. Overall, as shown in Figure 3, the MGEX orbit and clock products of Galileo presently cover a time span of almost 1.5 years, which enables long-term performance assessments under a wide range of conditions.
In the absence of published values for the GNSS antenna offset from the center-of-mass, conventional values of (x, y, z) = (+0.2, 0.0, +0.6)m have been adopted for the MGEX project and are recommended for the observation modeling when working with the MGEX orbit and clock products. These values refer to the orientation of the Galileo spacecraft coordinate system illustrated in Figure 4.
Similar to GPS, the IOV satellites employ a yaw-steering about the Earth-pointing z-axis to maintain the solar panel axis y perpendicular to the Sun-direction. Other than for GPS, however, the Sun is always maintained in the ‒x hemisphere, while the +x-panel carrying the atomic frequency standard is oriented towards the deep space. The article by A. Konrad et alia listed in Additional Resources discusses this subject in greater detail.
All MGEX analysis centers make use of an ionosphere-free combination of E1 (Open Service) and E5a observations in their Galileo processing, and the resulting satellite clock offsets apply specifically for this set of observations. For single-frequency processing or use of E5b and E5AltBOC observations, appropriate group delay parameters need to be considered. These can, for example, be obtained from the Galileo broadcast ephemeris message.
Figure 5 provides a comparison of Galileo IOV precise orbit estimates from TUM and CODE. Averaging all satellites over the eight-month analysis period, the two products exhibit a consistency of about 16 centimeters (3D rms position difference). This contributes an orbit-only uncertainty of roughly 5 centimeters to the user range error. CNES/CLS orbit products currently show a roughly three times larger error, which can largely be attributed to the use of one-day data arcs (as opposed to three- or five-day solutions provided by the other analysis centers).
For an independent performance assessment, we have used satellite laser ranging (SLR) measurements collected by the International Laser Ranging Service (ILRS). On average, some 50 normal points are collected per day for each of the four Galileo IOV satellites. Residuals of the SLR measurements with respect to the GNSS-based orbit solutions provide a direct measure of the line-of-sight orbit errors.
Figure 6 illustrates the results of this SLR data collection for satellite pairs IOV-1/2 (PRN E11/E12) and IOV-3/4 (E19/E20), which are located in orbital planes B and C, respectively. Aside from a mean radial bias of about 5 centimeters, which is as yet unexplained, the residuals show a distinct bow-tie pattern with peak amplitudes of about 20 centimeters and a standard deviation of about 8 centimeters. These values clearly exceed the self-consistency of the CODE and TUM orbit products and indicate the presence of highly correlated common errors in both solutions.
As may be recognized from a comparison of SLR residuals for individual satellites, the error amplitude is primarily related to the Sun angle above the orbital plane (i.e., the β-angle). The residuals are smallest whenever the Sun achieves its maximum elevation above the respective plane. However, a secondary minimum can be noted in the vicinity of the eclipse season, when the β-angle vanishes.
The radial orbit errors evidenced by the SLR residuals indicate a subtle error in the modeled accelerations. In view of their obvious correlation with the Sun aspect angle, a deficiency of the solar radiation pressure modeling presently appears as the most plausible cause of these errors.
Indeed both CODE and TUM employ the same 5-parameter ECOM model described in the article by T. A. Springer et alia (Additional Resources), which is well proven for GPS but appears to be less adequate for the Galileo satellites. Further analyses will be required to assess the potential benefits of a full-featured box-wing model or a ROCK-type a priori model as proposed in the presentation by D. Svehla et alia.
An overview of the Galileo IOV clock offset and drift based on MGEX precise ephemeris products is shown in Figure 7. Over the past year, the clock offsets have mainly been confined to less than 1.5 milliseconds with typical drifts of 5–20 microseconds per day relative to the GPS time scale.
Both hydrogen masers and rubidium clocks have been operated in an alternating manner on the individual IOV satellites. MGEX observations and clock products can be used to characterize the Allan deviation over a wide range of timescales; however a detailed clock analysis is beyond the scope of this paper and left to other publications, e.g., the article by A. Hauschild et alia.
QZSS. Next to Galileo, QZSS is the second emerging navigation satellite system for which precise ephemeris products are generated within the MGEX project. As of fall 2013, only one MGEX analysis center (TUM) determines precise QZSS orbit and clock data based on observations of the MGEX network.
However, final QZSS products generated by the JAXA control segment based on a mission specific monitoring network have been provided as a complement to the TUM products since August of this year. The contribution of a new multi-GNSS ephemeris product generated by JAXA with the new MADOCA software is foreseen in 2014 following dedicated inter-agency comparisons and completion of the software validation.
Figure 8 compares TUM and JAXA orbits solutions for QZSS for a one-month period in July/August 2013. The two products exhibit a consistency of about 0.7 meter (3D position difference), while the radial component agrees to roughly 0.1 meter. This is essentially consistent with satellite laser ranging residuals of about 0.15 meter for each of the two products in the same time period.
Although this comparison demonstrates a good performance of MGEX QZSS products during phases of high β-angle, a degraded quality may appear when QZSS attains an orbit-normal attitude for |β|<20 degrees. Here, the standard IGS yaw-steering attitude model no longer applies, and the parameterization of the radiation pressure must be adapted to account for the actual orientation of the spacecraft body and the solar panels.
BeiDou. The BeiDou system declared an operational regional navigation service in December 2012 and provides broadcast ephemerides of good accuracy to its users. Various Chinese institutions (such as Wuhan university) compute precise orbit and clock products making use of proprietary networks as well as MGEX monitoring stations.
For BeiDou satellites in medium Earth orbit (MEO) and inclined geosynchronous orbit (IGSO), overlap accuracies at the 10-centimeter level (3D position) and SLR residuals of similar order have been reported in the paper by Q. Zhao et alia (Additional Resources). BeiDou GEOs achieve a degraded performance with along-track errors of about 0.5 meter as a result of the static observation geometry.
Within the MGEX project, the generation of precise orbit and clock products for BeiDou has not yet started, but efforts are made to provide first such products in early 2014.
Broadcast Ephemerides
In an effort to provide users with orbit and clock information for all GNSS satellites presently tracked by the MGEX network, TUM and DLR generate a cumulative broadcast ephemeris file with GPS, GLONASS, Galileo, BeiDou, and SBAS information on a daily basis for the MGEX project. Overall, the file provides ephemerides of more than 80 satellites, and this number will increase as more satellites are deployed.
For improved compatibility with existing software packages, which may not have full-featured implementation of the broadcast orbit models for all constellations, the IGS will also provide an SP3 (National Geodetic Survey Standard GPS Format) version of the ephemeris file. In this context, an extension for the SP3c format accommodating more than 85 satellites has been initiated and is under review within the IGS.
Even though the accuracy of the broadcast orbit and clock information is not fully competitive with precise data products, the broadcast ephemerides are well suited for numerous applications, such as visibility analysis, quality control of observation data, or relative navigation.
In case of BeiDou, for which precise ephemerides are not yet available within the MGEX project, the broadcast ephemerides achieve a typical user range error at the 1.5-meter level. The broadcast ephemerides also provide access to timing and broadcast group delay parameters (TGD, BGD) and inter-constellation system time offsets for new constellations for which no alternative products are currently available in the MGEX project.
In view of the current limitation of the RINEX 3.02 navigation message format, the cumulative broadcast file is limited to legacy (L1 C/A-code) navigation messages for GPS and QZSS, but does not support CNAV and CNAV2 messages broadcast with the new L2C, L5, and L1C signals.
Nevertheless an effort was made within the MGEX project to collect CNAV broadcast ephemerides during the first GPS CNAV test transmission in June 2013 with a limited set of receivers. Aside from binary raw navigation data frames transmitted by the Block IIR-M and IIF satellites during the campaign, the decoded navigation and auxiliary data have been archived in a RINEX-style format and are made available to interested users through the CDDIS.
Future Work
The build-up of the MGEX network has laid the foundation for an early familiarization with new GNSS signals and systems. Even though first steps have been made to provide precise ephemeris products for individual constellations, a major effort still needs to be made before a comprehensive multi-GNSS service can be offered within the IGS.
Key activities to be pursued by the Multi-GNSS Working Group in cooperation with other IGS entities over the next year include:
- the expansion of multi-GNSS tracking capabilities within the frame of an overall IGS network
- the consideration of additional constellations (BeiDou, IRNSS and, optionally SBAS) in the precise ephemeris generation
- the development of a new multi-GNSS/multi-signal differential code bias product and its generation within the ionospheric data processing
- the characterization of the new GNSS satellites (antenna offsets and phase pattern, attitude modes, solar radiation pressure models, maneuvers) and the development of common processing standards for orbit and clock products
- the development of multi-GNSS/multi-signal quality control tools (noise, multipath, cycle slips, and so forth), and their routine application for monitoring of the overall network.
These tasks will help to pave the way for a comprehensive consideration of new navigation satellite systems in engineering and science and strengthen the role of IGS as a leading provider of free GNSS data and products of the highest quality.
Summary and Conclusions
Over the past one to two years a global multi-GNSS network has been established with initial products being delivered to the scientific community. Future work within the MGEX project focuses on the incorporation of additional constellations, the improved characterization of the space and ground segment, and the provision of new product types. Subject to active participation by MGEX analysis centers and a timely build-up of the necessary processing chains, the MGEX project is expected to transition into a multi-GNSS Pilot Project within the next couple of years.
Acknowledgment
This article is based on a paper presented at the 4th International Colloquium on Scientific and Fundamental Aspects of the Galileo System held in Prague, Czech Republic, December 4–6, 2013.
Additional Resources
1. Boriskin, A., and D. Kozlov, and G. Zyryanov, (2012),“The RTCM Multiple Signal Messages: A New Step in GNSS Data Standardization,” Proceedings of the 25th International Technical Meeting of The Satellite Division of the Institute of Navigation (ION GNSS 2012), Nashville, Tennessee USA, pp. 2947–2955, September 2012
2. GPS Directorate, Global Positioning System Modernized Civil Navigation (CNAV) Live-Sky Broadcast Test Plan, May 30, 2013
3. Hauschild A., and O. Montenbruck, and P. Steigenberger,“Short-Term Analysis of GNSS Clocks,” GPS Solutions 17(3):295-307, DOI 10.1007/s10291-012-0278-4, July 2013
4. IGS Multi-GNSS Experiment homepage, (alternate URL for access from China)
5. Ikari, S., and T. Ebinuma, R. Funase, and S. Nakasuka, “An Evaluation of Solar Radiation Pressure Models for QZS-1 Precise Orbit Determination,” ION-GNSS-2013, Nashville, Tennessee USA, September 2013
6. International GNSS Service, “RINEX – The Receiver Independent Exchange Format, Version 3.02,” IGS RINEX Working Group and RTCM-SC104, April 3, 2013
7. Konrad, A., and H.-D. Fischer, C. Müller, and W. Oesterlin, “Attitude & Orbit Control System for Galileo IOV,” 17th IFAC Symposium on Automatic Control in Aerospace, DOI 10.3182/20070625-5-FR-2916.00006, 2007
8. Langley, R.,“The Almanac,” GPS World, August 2013, pp. 47-50
9. MGEX Product Archive
10. Miyoshi, M., and S. Kogure, S. Nakamura, K. Kawate, H. Soga, Y. Hirahara, A. Yasuda, and T. Takasu,“The orbit and clock estimation result of GPS, GLONASS and QZSS by MADOCA,” 23rd International Symposium on Space Flight Dynamics, Pasadena, California, October 29–November 2, 2012
11. Montenbruck, O., and P. Steigenberger (2013a), “The BeiDou Navigation Message,” IGNSS Symposium 2013, Gold Coast, Australia, July 16–18, 2013
12. Montenbruck, O., and R. B. Langley, and P. Steigenberger (2013b), “First Live Broadcast of GPS CNAV Messages,” GPS World, Vol. 24, No. 8, August 2013, pp. 14-15
13. NASA Crustal Dynamics Data Information System (CDDIS), June 2013 CNAV Campaign Directory
14. Pearlman, M. R., and J. J. Degnan, and J. M. Bosworth, “The International Laser Ranging Service,” Advances in Space Research, 30(2):135-143 DOI:10.1016/S0273-1177(02)00277-6, 2002
15. Radio Technical Commission for Maritime Services, RTCM Standard 10403.2 Differential GNSS (Global Navigation Satellite Systems) Services – Version 3 with Amendment 1, RTCM, Arlington, Virginia USA, July 12, 2013
16. Springer, T. A., and G. Beutler, and M. Rothacher, “A New Solar Radiation Pressure Model for GPS Satellites,” GPS Solutions 2(3):50-62, 1999
17. Svehla D., and M. Rothacher, U. Hugentobler, P. Steigenberger, and M. Ziebart, “Geometrical Model of Solar Radiation Pressure Based on High-Performing Clocks Onboard new GNSS Satellites,” IAG Scientific Assembly, Potsdam, Germany, September 2013
18. Weber, G., and D. Dettmering, H. Gebhard, and R. Kalafus, “Networked Transport of RTCM via Internet Protocol (Ntrip)—IP-Streaming for real-time GNSS applications,” ION-GPS-2005, Long Beach, California USA, September 13–16, 2005
19. Zhao, Q., and Z. Hu, J. Guo, M. Li, X. Shu, G. Chen, C. Shi, and J. Liu, “Positioning Performance of BeiDou Navigation Satellite System,” IGNSS Symposium 2013, Gold Coast, Australia, July 16–18, 2013