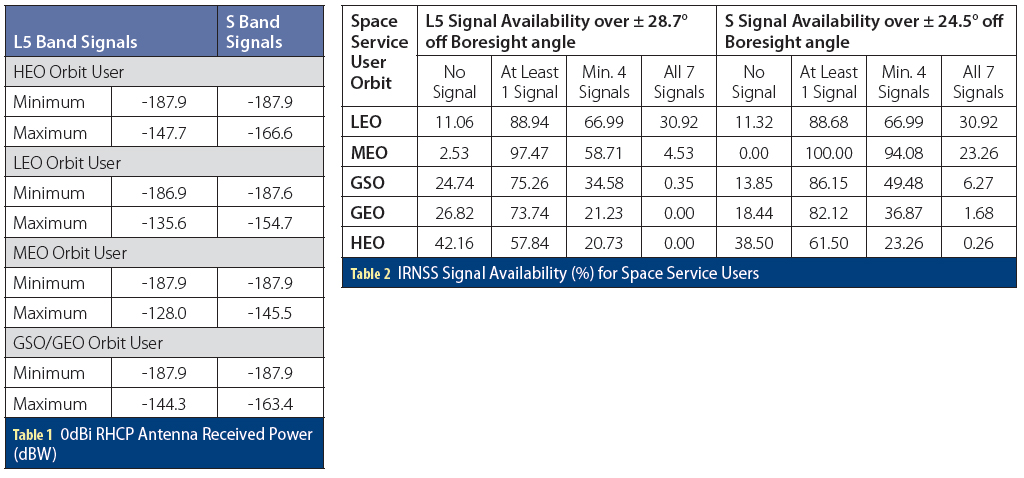
Present satellite-based navigation service providers are improving their services by adding new signals as well as by improving signal structure. Many countries are launching their own satellite constellations for navigation services, which will provide their services on earth and surrounding space. Today navigation operators are exploring the Space Service: Position, Velocity, and Timing (PVT) of other spacecraft equipped with navigation receivers. It is a totally new utility being developed beyond its original purpose of providing PVT services for land, maritime, and air applications.
Earth and space weather prediction, space vehicle formation flying, earth and space science research, exploration missions to the Moon and beyond, as well as military applications will all benefit from these new capabilities.
In the future, GNSS will have two types of service regions: Terrestrial Service Volume (TSV) and SSV. The TSV can be viewed as a shell that begins at the surface of the earth and up to 3,000 kilometers altitude. The transmitted position finding parameters are valid for the entire region. That means the PVT performance will remain same for all users in this region. Users in the TSV have coverage from the main beams of the satellite antenna.
The SSV is a shell extending from 3,000 kilometers altitude to approximately the geostationary altitude, that is 36,000 kilometers. The SSV is further subdivided into two regions: 3,000 kilometers to 8,000 kilometers, and 8,000 kilometers to 36,000 kilometers (as described by F.H. Bauer et alia, in Additional Resources). The space users (SU) will have a different level of performance as per altitude. Within the SSV, nearly all navigation signals emanate from satellites across the limb of the Earth. Users may experience periods when no navigation satellites are available and received power levels will be weaker than the terrestrial users (TU). Timing correction for SU need to be provided. Figure 1 (see photo at the top of this article for all figures) shows the shells of different service regions. The following sections will describe the NavIC constellation, as well as detailed analysis and simulation results of NavIC space service availability, signal strength and other related details.
IRNSS/NavIC Constellation
The IRNSS has been established for regional navigation services over India using a combination of GEO and GSO spacecrafts.
The NavIC constellation consists of seven satellites — three Satellites in GEO orbit (at 34 degrees E, 83 degrees E and 131.5 degrees E) and four Satellites in GSO orbit inclined at 29 degrees to the equatorial plane with their longitude crossings as 55 degrees E and 111.5 degrees E (two in each plane) as shown in Figure 2 (see P. Majithiya et alia, Additional Resources). All the satellites are visible over the Indian region for 24 hours.
The IRNSS System is expected to provide two sigma position accuracy of better than 20 meters over India and a region extending to about 1,500 kilometers around India.
The IRNSS provides two types of services i) Standard Positioning Service (SPS) and ii) Restricted/Authorize Service (RS). Both of these services will be provided at two frequencies — one in the L5 band and the other in S-band.
IRNSS/NavIC Space Service
The antenna beam pointing location (boresight) of all IRNSS satellites is 83 degrees, E 5 degrees N. The nominal received power for the L5 terrestrial user is -157.8 decibel/watts (dBW) over its service region. The simulations have been carried out to find out the signal availability of IRNSS signals for different satellite orbits i.e., HEO, Geo Synchronous Orbit (GSO), GEO, MEO and Lower Earth Orbit (LEO). The GEO and GSO height satellite orbits are more suitable for this type of service, as it provides better coverage and availability. The coverage will be over ± 28.7 degrees (L5 signal) and 24.5 degrees (S Band signal) off boresight angle for each satellite as shown in Figure 3. The following sections discuss various system parameters for space navigation service.
NavIC Space Service Volume (SSV)
IRNSS GEO and GSO constellations can provide navigation services to the space users in LEO, MEO, HEO and GEO. All satellite antenna beams are pointed towards the center of the NavIC service area. The maximum transmit power will be available to the terrestrial user at the beam center of the antenna. These satellites are also emitting power from its main as well as side lobe across the limb of the Earth. Based on the IRNSS antenna gain pattern, the coverage considered for space service is ± 28.7 degrees and ± 24.5 degrees off boresight angle for L5 and S band signals, respectively.
Received Signal Power Level
The primary service of all navigation constellations is terrestrial so they focus major signal power on the earth surface. Because of this, the signal power will be less to the space user compared to terrestrial user at the edge of the main lobe or the side lobe of the antenna gain pattern. The signal strength will also vary for various satellite orbits. The minimum received power is measured by simulation at the output of a 0 dBi (decibels relative to isotropic) right-hand circularly polarized user receiving antenna. The power provided in the Table 1 (see inset photo, above right, for tables) are at worst orbital location with normal orientation of the user, at the off nadir angles.
The antenna radiation gain pattern is considered up to off-boresight angle ± 28.7 degrees for L band signal and ± 24.5 degrees for S band signal. The minimum and maximum received power at 0dBi gain receive antenna output were determined by simulating different orbital users over 1,148 grid points for each orbital user as shown in Figure 4.
The power given in Table 1 is the worst case power of any orbital user out of any IRNSS satellite. The power variation range (from its nominal received power of -157.8 dBW for terrestrial user) for different orbital users are approximately less than 60 decibel and 42 decibel, respectively, in L5 and S band signals.
Satellite Availability
The number of satellites decide the service availability and its performance. In space service navigation, at least one satellite signal should be available to any type of orbiting user to maintain the system time. As satellite visibility is an issue of geometry and statistics, the simulation is carried out based on grid sample points — 1,148 at each altitude as shown in Figure 4. In fact, user height is the dominant variable that determines GNSS satellite visibility in the SSV. Based on simulation of the NavIC constellation, the signal availability has been determined. The percentage of L5 and S band signals availability for no signal, at least one signal, four signals and all seven signals is shown in Table 2. Figure 4 shows the simulation for different locations of the user over an orbit. L5 band users in LEO orbit will get a minimum four satellite signals availability at 66.99% of the area at the altitude of 1,000 kilometers, and GSO orbit users will get four signals availability at 34.58% of the area at the altitude 36,000 kilometers. S band users in LEO orbit will get minimum four satellite signals availability at 66.99% of the area at the altitude 1,000 kilometers, and GSO orbit users will get four signals availability at 49.48% of the area at the altitude 36,000 kilometers. HEO (maximum 70,000 kilometers altitude) orbit satellite users will get lesser four signals availability compared to MEO and GSO signals, that is 20.73% and 23.26% of the area at the altitude 70,000 kilometers, respectively, in L5 and S band signals. IRNSS constellation will provide good availability for at least one signal for HEO users, that is 57.84% and 61.50% of the area at the altitude 70,000 kilometers, respectively, in L5 and S band. MEO orbit users will have very good signal availability for four satellites, that is 58.71% and 94.08% of the area at the altitude 25,000 kilometers, respectively, for L5 and S band services. GEO users will get the lowest number of at least one and four number of signals values (as shown in Table 2), but this is still better than MEO GNSS constellation. These availability figures show that the IRNSS constellation will play major role in this service.
Today, the major goal for the space vehicles in the MEO and GSO/GEO volume is to maximize the availability of navigation signals, with four satellites always in view to ensure robust navigation performance within the coverage. The major goal for space vehicles in the HEO orbit is the availability of at least one navigation signal at all times. This ensures precise on-board timing, at all times within the HEO volume, reducing the need for very expensive clocks on-board. Combining GPS and Galileo would enable an average of three satellites in view at GEO, with four satellites in view 30 percent of the time. Using all constellations (GPS, Galileo, GLONASS, BeiDou, QZSS, and NavIC) would enable four satellites visible at Geo approximately 95 percent of the time using the signals in the L1 frequency band (see J. Miller et alia, Additional Resources).
Signal Group Delay Parameters
The navigation signal transmission antenna in IRNSS is a helix array antenna, which will have amplitude and phase contour for off-nadir angle. The delay variation depends on off-nadir angle and frequency, so there is phase contour which gives delay difference for space navigation users with respect to terrestrial users. This delay bias, hereafter called Space User Delay (SUD), will be measured at the time of payload testing (see again P. Majithiya et alia, Additional Resources). The SUD will give additional accuracy to the space users who are using the signal over the limb of the earth at the off-nadir angle of greater than 8.4 degrees with respect to the IRNSS satellite. This bias term is frequency specific and satellite specific.
The SSPS single frequency space user should use the following equation to get better accuracy:
(ΔtSV)S-SPS = ΔtSV − TGD − SUDS (1)
Where TGD is provided to the user in the first subframe of navigation data.
TGD = (tS-SPS − tL5-SPS) × 1/(1 − γSL5)
Where, γSL5 = Ratio of S and L5 Band Squared Frequency: (fS/fL5)2 = (2492.028/1176.45)2
The L5SPS single space user should use the following equation to get better accuracy:
(ΔtSV)L5-SPS = ΔtSV − γSL5TGD − SUDL5 (2)
The typical navigation payload delay performance for worst case difference w.r.t. nadir is of the order of 3nsec for SSV users.
Ionosphere Error Correction
The SV clock offset estimation reflected in the af0 clock correction coefficient is based on the effective PRN code phase as apparent with two frequencies (SSPS and L5SPS) and ionosphereic error corrections. Thus, the user employing both L5 and S in the ionosphereic error correction need make not further correction.
Space users should use the following equation to get ionosphere error correction:
PR = ((PRL5-RS − γSL5PRS-RS) + c(SUDL5 − γSL5SUDS)) / (1 – γSL5) (3)
Where,
PR = Pseudo range corrected for ionosphereic effect
PRL5-SPS/S-SPS = Pseudo range measured on the L5SPS or SSPS frequency channel, SUDL5/S = Space User (payload hardware)
Delay for L5 and S band signals c = Speed of light
User Range Error (URE)
URE is the error bound on range measurement. This is a function of accuracy of orbit and clock solutions from ground segment, Age of Data and Uncertainty in physical and modeling parameters such as antenna group delay and phase center variation as a function of off-nadir angle. The current targeted URE requirement by the GNSS community is ≤ 0.8 meter (rms) for this SSV service.
Space User Receiver Requirement
A navigation receiver design relies on the assumption of good signal visibility and high signal strength, neither of which are available when we try to use them in space service volume. At high altitudes in addition to presence of extreme signal dynamics, the power levels are weaker, signal visibility is sparse and geometries are poorer. Heritage navigation receivers cannot be directly used in SSV because of differences in vehicle dynamics, signal levels and geometrical coverage.
The space user receiver will receive signals from the edge of the main lobe or from the side lobe so the signal strength will be less when compared to terrestrial service. Therefore, the receiver should be very sensitive and it should work at low power signal. Space borne receivers have to handle strong signal dynamics during their orbital flight, which require adaptive signal processing algorithms and dedicated strategies for satellite search and selection. In addition, accurate orbit propagation and dynamic models to propagate the vehicle state estimate is essential for navigation receivers to be used at high altitudes. Furthermore, capabilities to support the tracking of navigation satellite through multiple antennas in multiple orientations should be explored in addition to the use of earth pointing high gain antennas. Also the components with sufficient level of radiation tolerance should be considered. Due to power constraint, rapid cold start capability, fast acquisition, satellite selection and tracking of all available signals when the carrier-to-noise density (C/N0) reduces should be considered.
The recovery from temporal jamming condition needs to be addressed when the receiver in HEO orbit comes in close proximity to one of the satellites. The most fundamental requirement for an HEO receiver is a robust navigation filter and clock model to enable operation when fewer than four satellites are visible simultaneously. One of the major requirements of user receiver for space service application is that it should be multichannel and multi constellation.
Conclusion
Navigation space service is an immerging application. It is a challenge for any GNSS service provider to cover full space service volume so compatibility and interoperability with other GNSS signals are a must. The NavIC SSV will be over ± 28.7 degrees and ± 24.5 degrees off boresight angle for L5 and S band signals, respectively. Simulation shows that the GEO/GSO constellation provides very good availability of a number of signals with reasonable received power. IRNSS signals are also available to GEO orbit user with good received power. This paper addresses the contribution of NavIC in this service so that in the future space users can plan for a suitable receiver. NavIC constellation can be used for a full range of Earth orbiting missions from LEO to GEO and beyond for both scientific and commercial programs.
Acknowledgements
The authors are grateful to Shri. Tapan Misra, Director, Space Applications Centre (SAC), Ahmedabad and Shri D K Das, Associate Director, SAC for providing overall guidance and encouragement to carry out this work.
Additional Resources
[1] Bauer, F.H., M.C. Moreau, M.E. Dahle-Melsaether, W.P. Petrofski, B.J. Stanton, S. Thomason, G.A Harris, R.P. Sena, L. Parker, “The GPS Space Service Volume”, Proceedings of the 19th International Technical Meeting of the Satellite Division of The Institute of Navigation (ION GNSS 2006), Sep. 2006.
[2] Majithiya, P., K. Khatri, J. K. Hota, “Navigation Satellite System Correction Parameters for Timing Group Delays”, Inside GNSS, January/February 2011, Vo. 6, No. 1.
[3] Miller, J., F.H. Bauer, J. Donaldson, A. J. Oria, S. Pace, J. Parker, B. Welch, “Navigation in Space, Taking GNSS to New Heights”, Inside GNSS, November/ December 2016, Vol. 11, No. 6.