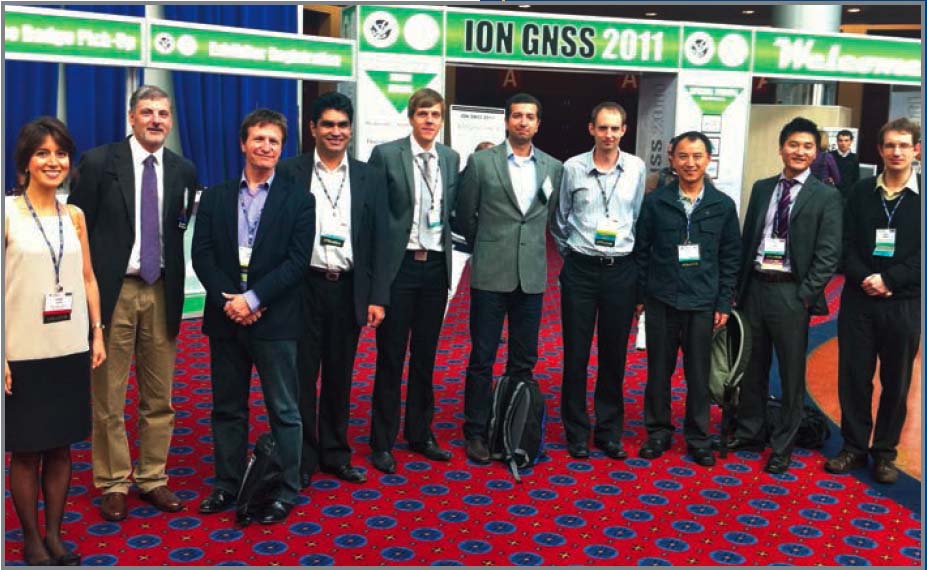
The diversity and redundancy provided by multiple, independent, compatible, and in some respects, interoperable GNSS systems must be a good thing, right?
Well, almost certainly. But as with many things in life and technology, the devil’s in the details. And, as the varied characteristics and design specifications of new GNSSes and regional systems become clearer, it may not be too early to sort out those details.
The diversity and redundancy provided by multiple, independent, compatible, and in some respects, interoperable GNSS systems must be a good thing, right?
Well, almost certainly. But as with many things in life and technology, the devil’s in the details. And, as the varied characteristics and design specifications of new GNSSes and regional systems become clearer, it may not be too early to sort out those details.
Poll a representative cross-section of experts, and a consensus forms around the top three benefits of using signals from more than one GNSS: increased accuracy, integrity, and service availability — with continuity of service running a close fourth.
In challenging environments, such as urban areas, many signals are contaminated by non-line-of-sight reception or multipath interference. With multi-constellation GNSS, the best signals may be selected for a navigation solution and the worst ones discarded.
Moreover, multi-constellation GNSS brings a significant improvement in the proportion of space and time over which sufficient signals are available without interruption to compute a position solution.
Signal diversity and redundant measurements, together with better geometry from multiple GNSS satellites, allows improved receiver-based integrity monitoring to be carried out, including the detection of multiple satellite/signal failures.
These terms are equally applicable across all domains. For example, when sensing the atmosphere in order to estimate tropospheric delays (which feed into weather forecasting and climate-change studies), the increased number of signal rays through the atmosphere greatly improves the ability to refine the temporal and spatial density of the derived parameters and models.
Beyond this terra cognita, however, more systems bring more variables and more choices, and the way ahead is not always clear.
To help illuminate the unknowns, we called on not one expert, but rather a gallery of them from the iNsight Project — “Innovative Navigation using new GNSS SIGnals with Hybridised Technologies.” Four leading British academic GNSS research centers comprise iNsight.
Participants in this virtual roundtable included the following (with the initials by which they are identified in the answers to our questions): Professor Terry Moore (TM), Director of the Nottingham Geospatial Institute at the University of Nottingham; Professor Marek Ziebart (MZ), Head of the Space Geodesy and Navigation Research Group at University College London (UCL); Dr. Paul Groves (PG), a lecturer in the same group at UCL; Professor Washington Ochieng (WO), Director of the Imperial College London (ICL) Engineering Geomatics Group; Dr. Shaojun Feng (SF), an ICL research fellow; Professor Izzet Kale (IK), Director of the Applied DSP and VLSI Group at University of Westminster.
IGM: What are the practical benefits for product designers and system integrators of having common frequencies and signal designs for GNSS signals?
iNsight: This significantly simplifies RF front-end design at the expense of a slight increase in susceptibility to inter-system interference. The antenna, front-end, and correlator design can be much simpler compared to components designed to operate with multiple systems, and in particular the filtering within the front end of a receiver can also be tighter. A receiver processing signals from different constellations sharing the carrier frequency does not require multiple RF and IF stage filters. The digital front-end, delivering the signals to the baseband processor, would also be simplified. The similarity of the different systems means that signals can be processed in a similar way. Obviously, the positioning algorithms will need extension to cope with the diversities of multiple systems. Beyond this, common signal designs only reduce software development costs. (IZ, TM, PG, WO, SF)
IGM: What benefits can common time and coordinate systems among GNSS systems bring to GNSS users and equipment manufacturers?
iNsight: Clarity in performance metrics and in standardization. At present we have a plethora of potential coordinate systems/datums from which to choose. For the manufacturer this is not an issue since as long as a reliable transformation exists from one frame to another then a positioning solution can be provided. However, the user does care about coordinate systems and the quality of service delivered. Trends in aviation and the maritime industry show a steady dawning of awareness, followed inevitably by a movement towards a single global datum for positioning and mapping products.
Time is often the elephant in the error budget for GNSS integration. For the mass market, this is largely unimportant. However, for the system developer the issue is becoming more complex. With a choice of frequencies, we have a choice of timescales based on multiple possibilities for ionosphere-free clock solutions. Discontinuities in one timescale become observable against another. Service providers themselves may find they question their approaches. Even at this early stage of GPS/GLONASS integration the optimal solution is not apparent. Class! More work required! (MZ)
IGM: From a technical as well as practical perspective, what are the most promising non-GNSS technologies for integration with GNSS?
iNsight: The time is probably right for a change of positioning and navigation philosophy. The question should no longer be which technologies should integrate GNSS. This may seem heresy in this publication, but perhaps we should now consider the inertial measurement unit (IMU) to be the primary positioning and navigation sensor, and the real question is what do we integrate with the IMU to bound the growth on the INS errors; GNSS, of course, being a prime candidate in most, but not all environments. (TM)
The benefits of integrating GNSS with dead-reckoning technologies are well known. As time progresses, the mix of technologies used for positioning will expand. Three new approaches currently emerging are signals of opportunity (SOOP), 3D mapping, and vision. SOOP comprises signals designed for purposes other than positioning, such as phone signals, GSM, WCDMA, Bluetooth, WiFi, WiMAX, and television. City models (3D mapping) enable new positioning techniques, such as shadow matching that offer improved accuracy in poor-geometry environments, such as urban canyons. It can also be used to identify reflected signals and for conventional map matching and height aiding. Digital cameras are cheap and becoming increasingly ubiquitous. They can be used to aid positioning in many different ways: measuring the distance travelled, position fixing through landmark identification, and aiding GNSS signal selection by detecting obstructions. SOOP should also include signals from low-earth-orbiting (LEO) communication satellites, because in remote mountain area where the GNSS reception is poor, the only SOOP maybe the signal from LEOs. (IZ, PG, WO, SF)
One in a series of articles sponsored by NovAtel Inc.