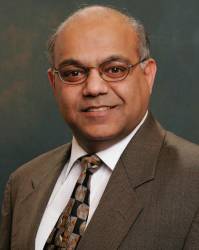
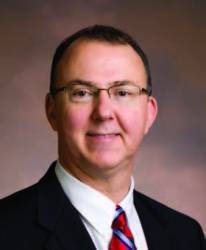
GNSS receivers seem to get all the attention. Go to any technical GNSS conference and the lion’s share of presentations are about receiver design and techniques: better algorithms, signal processing, integration with other sensors, spoofing detection, and on and on.
GNSS receivers seem to get all the attention. Go to any technical GNSS conference and the lion’s share of presentations are about receiver design and techniques: better algorithms, signal processing, integration with other sensors, spoofing detection, and on and on.
But here’s a fundamental fact of radio science: without antennas, GNSS receivers are essentially useless. Antennas are the component that picks up the GNSS signals out of the RF noise and channels them to the receiver proper — the better the antenna, the better the signals that receivers have to process.
Moreover, largely constrained by the laws of physics, the physical aspects of antennas play a substantial role in the size, weight, and power parameters within which receiver designers must work. And, by extension, antennas are a key variable in the cost factors associated with receiver manufacturing.
With these factors in mind, we turned to Dr. Inder “Jiti” Gupta for insights into the current state of GNSS antennas and their role in GNSS positioning, navigation, and timing. Currently a research professor with the Department of Electrical and Computer Engineering of The Ohio State University, Gupta has focused on GNSS antennas and antenna electronics for the past 17 years. An Edmond S. Gillespie Fellow of the Antenna Measurement Techniques Association (AMTA) the recipient of the 2007 AMTA Distinguished Achievement Award, he has authored more than 150 journal and conference papers.
IGM: What changes are taking place in the GNSS operational environment that pose increasing challenges for successful PNT applications?
GUPTA: Many changes are taking place, including increased GNSS operation in dense urban environments, inside buildings, on platforms that change rapidly with time, e.g., rotorcrafts. The major challenge, however, is posed by spectrum crowding and radio frequency interference (RFI) that could be intentional or unintentional. Spectrum crowding will lead to high-energy signals next to GNSS frequency bands and will require filters with very narrow passband and very high rejection ratio outside the pass band.
One will be looking at brick wall type of filters that are not only costly but can distort the signals of interest (satellite signals). RFI is within the GNSS signals frequency band and cannot be filtered in the frequency domain without affecting the satellite signals. Other approaches need to be applied for successful operation of GNSS receivers under strong RFI environments.
IGM: Can improved receiver antenna design help in these operational environments?
GUPTA: Yes. Currently, fixed-reception-pattern antennas (usually a single element and single feed) are used with GNSS receivers. As the name indicates, the response of these antennas does not change with the RF environment. If we replace these antennas with multiple element antennas whose weights can be controlled (adapted) in real time, then we can easily obtain spatial and polarization discrimination. For example, the signals received by various antenna elements can be combined to increase the gain along selected GNSS satellites.
One can also adapt the element weights to steer antenna nulls along the sources of RFI. Note that controlled reception pattern antennas (CRPA) used with many military GNSS receivers carry out null steering. One can combine beamforming with null steering to increase the antenna gain along the satellite direction while suppressing the RFI simultaneously. One can form ring nulls to suppress multipath or RFI originating around the horizon.
For applications where it is not possible to install antennas with multiple elements, one can use multiple feeds with a single aperture (microstrip patch antennas) and use the output of these feeds to carry out null steering and/or polarization discrimination.
IGM: What innovations in receiver/antenna software seem most promising?
GUPTA: I do not know if we can call it innovation or not, but array signal processing is one area that has not been exploited by GNSS receiver designers. Only recently has the navigation community started using this powerful technology to enhance the receiver performance in strong multipath and RFI environments and to geolocate the sources of interfering signals. With the advancements in field programmable gate arrays (FPGAs), we need to incorporate array signal processing in GNSS receivers.
IGM: Some application developers and handset manufacturer have expressed interest in implementing multi-GNSS capability in consumer products. What should be the considerations for antenna design & development to support the implementation of such capability?
GUPTA: A GNSS antenna is supposed to have omnidirectional (for handheld receivers) or upper hemispherical coverage (for mounted receivers). Thus, these antennas should be low directivity antennas. Also, a GNSS antenna should be an efficient antenna.
Two main factors dictate the antenna efficiency. First, how well is the antenna matched to the receiver? This is also called the return loss of the antenna (S11 parameter). A good number to shoot for is better than 10 decibels over all the frequency bands. The second factor is the radiation efficiency of the antenna which tells us how much of the incident RF energy antenna passes to the receiver. A good number to shoot for is better than 75 percent radiation efficiency over all frequency bands.
Another parameter to consider during the design and development is the antenna polarization. GNSS signals have right hand circular (RHC) polarization. For the best performance, GNSS antennas should have RHC polarization over all the frequency bands and field of view, which is upper hemisphere for mounted receivers and whole sphere for handheld receivers.
IGM: What challenges does one face in designing antennas for handheld multi-GNSS receivers?
GUPTA: For handheld GNSS receivers, major challenges are size and weight. The current commercial handheld GNSS receivers use GPS L1 C/A coded signals or maybe an L1 band GLONASS signal. The bandwidth of these signals is approximately two megahertz at 1575.42 MHz. The percentage bandwidth, thus, is very small.
It is easy to design a lightweight antenna with small volume for small percentage bandwidth. As the bandwidth increases, it becomes more and more difficult to make the antenna small without losing its efficiency. Either one has to use multiple antennas to cover all the frequency bands or use frequency independent antennas (spiral type antennas). In both cases, one will need more real estate, and that is a challenge for handheld GNSS receivers. In this case, one may want to consider wearable antennas for multi-GNSS receivers.
IGM: What are the relative strengths and weaknesses of anechoic chamber measurements versus real-world trials for testing of GNSS antennas?
GUPTA: Let us start with the weakness. The major weakness of anechoic chamber measurements is that it is difficult to simulate the real-world physical environment. Let us say that we are interested in measuring a GNSS receiver antenna mounted on a large SUV. I do not know many anechoic chambers that are large enough to measure antennas mounted on large SUV at GNSS frequency bands. Also, it is hard to duplicate the surroundings.
On the other hand, anechoic chamber provides a very controlled RF environment. One can choose what signals to be simulated and the relative strengths of those signals. For example, one can transmit very strong (more than 20-decibel signal-to-noise ratio) signals in GNSS frequency bands to measure the antenna response (gain and phase) at those frequencies. Thus, one can obtain very accurate antenna response without very long integration. One can also sweep the frequency to cover the whole frequency band of interest. With current technology, frequency sweep is trivial and extremely fast. One can cover the whole L-band in a few seconds. Also, using two independent motion controls, one can control the attitude of the antenna under test to measure its response over the whole field of view. Thus, anechoic chambers are well suited to verify the antenna design by measurements.